Trees Are Dying at Shocking Rates — Understanding How Could Ultimately Save Them
To predict the fate of the world’s woods in the face of climate change, researchers need to understand how trees die.
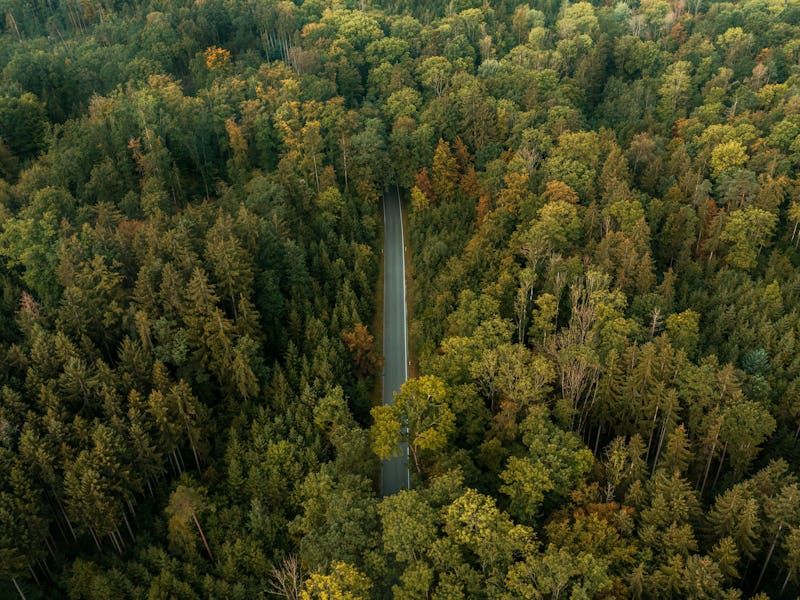
As we hang far above the ground on a sunny October day, it would be easy to focus on the blue crests of hills and the small towns tucked in between. But Richard Peters, who’s with me inside a metal gondola mounted onto the maneuverable arm of a crane, points me instead to the tree canopy below, flushed with the gold and copper shades of fall. “That guy is definitely on his way to die,” he says of one tree.
We hover over the leafless, barren branches of a beech tree that lost its crown due to drought, a spruce with a tip stripped bare of needles, and, in the distance, we see the bald skeletons of conifers ravaged by bark beetles.
Peters yells instructions, and the man operating the crane steers the 50-meter-long arm around in a circle, allowing the gondola to glide over the forest roof as a breeze gently tickles the leaves. It’s a surreal way to view the forest canopy, and for Peters and the other scientists who work here, it’s a lot more than that. They regularly visit this grove in Switzerland’s Hölstein area in the Jura Mountains to take meticulous measurements from roughly 80 of the 480 trees, right in the zone where they breathe.
Some 14 European tree species, mostly beech and spruce, grow here, the subject of a long-term study led by plant ecologist and physiologist Ansgar Kahmen of the University of Basel. When the project was launched in 2018, the goal was to simulate the effects of drought by building roofs just above the forest floor to intercept the rain. But that summer and early fall, the weather itself set up the experiment, with rainfall slashed nearly in half and temperatures three degrees higher than usual as part of the worst drought to sweep central Europe in 250 years.
Many trees were wiped out; 10 spruce succumbed at the two-hectare site (about five acres). Countless other trees were tested to their limits that year and in the years since.
Forest scientists around the globe are alarmed to see droughts, often exacerbated by fire and infestations of bark beetles, cull trees at scales they have never seen before — from massive swaths of American woodland to dry forests in Australia where roots can reach down some 50 meters (more than 160 feet), to temperate regions and moist tropical forests where such events were long deemed unthinkable. “Even people who are really knowledgeable and who have a lot of experience out in the field were surprised to see how fast these forests were going down the drain,” says Henrik Hartmann, an ecophysiologist at the Julius Kühn Institute Federal Research Centre for Cultivated Plants in Germany and lead author of an overview of forest die-offs in the 2022 Annual Review of Plant Biology.
Droughts have struck many of these ecosystems before, but what’s different now are the supercharged “hotter droughts” brought on by sizzling temperatures. And more dramatic forest die-offs are on their way, warn Hartmann and his colleagues. Working out how many of these culls will strike and where is critical; forests are vital homes to terrestrial life and act as planetary air conditioners by absorbing up to one-third of the planet-warming fossil fuel emissions humanity produces annually. Some experts predict that if tree die-offs gather pace and expel more carbon into the air, forests could become net producers of carbon dioxide, accelerating climate change.
But forecasting the future is a mammoth challenge — so much so that the major climate predictions of the Intergovernmental Panel on Climate Change (IPCC) are likely to heavily underestimate drought-driven tree die-offs. Scientists don’t even know how many trees are dying in the present day; they largely only record deaths at well-studied sites, so many are probably dying unnoticed.
And importantly, much of the scientific understanding of how trees respond to drought is outdated, based on an incomplete consideration of tree physiology, making it difficult to build accurate models. Predicting what the future holds means untangling the silent processes unfolding inside the bodies of trees as they suffer through hotter and drier weather and — ultimately — understanding how trees die.
Watching drought impacts in action
It may seem odd that scientists still don’t know exactly how droughts kill trees. Then again, they rarely get the chance to track the death of a tree from start to end since it can take years, even decades, for a tree to succumb, and many inch closer and closer to the brink unnoticed.
That’s partly what makes the monitoring site in Hölstein so valuable. If a tree dies in this forest, scientists will hear it loud and clear.
After driving up a windy road off a highway near Basel, I arrive before dawn at the gated site on a mid-October Thursday, together with Peters, a tree physiologist at the University of Basel, and David Steger, who is doing a PhD at the same institution on below-ground drought responses. We don helmets, partly to protect ourselves from falling beech branches damaged by drought, and the scientists swing into action. Steger searches for the conifers to be inspected today and shines a flashlight up their trunks so that ecophysiologist Günter Hoch, high above in the gondola, can maneuver toward the canopies to collect twig samples.
Squinting through the darkness, I see instruments wired to trees to regularly monitor their vitals: the shrinking and swelling of bark tissue as the trees drink, the movement of sap from roots to leaves, and the overall girth of the trunk. The contraption-laden trees give the impression of a big, outdoor ICU.
Hoch drops down a bag full of twigs, and Peters and I head to a small cabin to take a key measurement from them: the leaf water potential, an indicator of how stressed a tree is. Leaves are studded with little valves called stomata, through which trees shuttle carbon dioxide and oxygen and allow water to exit; just like sweating, this water loss cools the tree. When water exits, it generates a negative pressure that sucks more water up through the xylem — the water-shunting channels inside the trunk and branches of a tree — toward the leaves. We’re measuring that negative pressure, also called tension.
Leaf water potentials are generally negative, but the less negative they are, the better. When measured before dawn, they show whether trees were able to rehydrate overnight, replenishing the water they lost the day before. That’s why I am here at 5 a.m., watching as Peters assesses the state of twigs of Norway spruce (Picea abies), silver fir (Abies alba), and Scots pine (Pinus sylvestris). He pokes each twig into an airtight chamber and slowly lets in pressurized air until sap bubbles erupt out of the cut end of the twig. The amount of pressure that’s needed for that to happen equals the water tension the twig was experiencing.
Peters seems pleased to find that the twigs’ water potential values are relatively high, in the range of -0.6 to -0.7 megapascals. Thanks to recent rains, the trees had recovered from the year’s punishing summer, where their leaf water potentials had plummeted to -2 megapascals because the trees were dehydrated. “They’re quite happy trees,” Peters says.
In the brutal summer and fall of 2018, the scientists watched as the daytime water potentials in the branches of 10 spruce trees they were tracking in the forest fell below -2.3 megapascals. Evidently, the drought, accompanied by a heat wave, had pushed the trees to their limits. Warm air can hold exponentially more water than cooler air can, leading to a situation where, degree by degree, more moisture is tugged out of the stomata and the tree as a whole. Trees can close their stomata to stall this water loss, but some water still escapes.
Once the roots ran out of water, the team believes, the spruce began to dehydrate, as they depleted their internal water reserves and lost water through their needles. The strain on the trees’ water columns became so great that liquid water vaporized, creating air pockets called embolisms that clogged up the xylem. If a tree has too many embolisms, the entire water transport system will fail to supply water to the canopy when soil moisture once again becomes available, Kahmen says — which is what happened to five spruce whose water potentials had tanked below -7 megapascals. They died of hydraulic failure — essentially, of thirst. “The processes that we observed in Norway spruce were quite remarkable and unseen before,” Kahmen says. “It is rare to show that hydraulic failure is really the sole mechanism for mortality.”
The findings, published in 2021, suggested that Norway spruce are much more vulnerable to drought than had previously been thought — which is a problem since the tree is planted over much of Europe for timber. The work also fed into a heated debate about how exactly drought kills trees. Although hydraulic failure is often thought to be the fatal blow — and certainly was for the Norway spruce — some scientists argue that drought can first cause trees to starve. Trees burn through their energy reserves faster at warmer temperatures because their metabolisms rev up. And if they’ve shuttered their stomata to protect themselves from water loss, they can’t cool themselves or take in as much of the carbon dioxide they need to photosynthesize and make sugars for essentials like metabolism, water uptake, and repairing embolisms. It is a vicious cycle that, in turn, makes them more prone to hydraulic failure.
How much of it boils down to starvation versus dehydration? Or does it depend on the species? Getting an answer is not unlike deciphering the ultimate cause of death in people who have multiple, intertwining health conditions, says Alana Chin, a tree ecophysiologist at ETH Zürich. “This is part of why we are surprised by these tree mortality events because we’re not totally sure how it works.”
Tricks of survival
Death and dying is the end of the road. But an equally important question is what makes trees susceptible to drought to begin with. Many trees have tricks to stave off dangerous levels of dehydration and embolisms. Scots pine, a scrappy-looking, slow-growing conifer, shutters its stomata quickly — at least compared to spruce. Its tough needles help to prevent water from escaping; its often slightly skinnier xylem channels may make it harder for embolisms to develop; and stored water in its bark tissue helps it to persevere through dry times. “This is basically a species that holds its breath,” Peters says.
Norway spruce, by contrast, prioritizes photosynthesis and rapid growth at the cost of safety; it’s lazier about closing its stomata and has less water storage capacity in its trunk.
European beech, a relatively fast-growing species, is also drought-sensitive, but it can shed its leaves to prevent losing water through its stomata, and during severe droughts, it can slough off entire branches.
But stomata and xylem aren’t the whole story. During the 2018 drought, Kahmen and his colleagues noticed that some species did remarkably well even though they left their stomata wide open. That includes oaks and what Peters proudly calls the Basel site’s “supertree”: Sorbus torminalis, also known as the wild service tree. The secret of these trees, the team suspects, may be long roots that haul water from deep soil layers that species like beech and spruce can’t reach, keeping water potentials steady and photosynthesis going. As long as trees have a straw in water, they’ll be fine, Hoch says.
Other factors, some not understood, also affect a tree’s fate during drought. European beeches, for example, are suffering in Hölstein, but it’s Steger’s impression that they’re doing better in a drier area near Berlin — perhaps because they are rooted more deeply there. Those hardy Scots pines are faring well in Hölstein’s loam soils, but drought has been killing them in droves in other parts of Switzerland on sandier, faster-draining terrain.
It’s shocking to witness, Chin says. “Seeing Scots pine, of all things, dying from apparent water stress and on a really large scale … it’s really not something that’s been seen before.”
Diagnosing forest die-offs
Across the world, scientists have been similarly caught off guard by the effects of hotter droughts on forests that were thought to be resistant.
In 2015, a delayed and meager rainy season caused hundreds of trees to die in a forest in Guanacaste in northwestern Costa Rica that periodically cycles between wet and dry seasons. Again, the most severely affected species were the most vulnerable to hydraulic failure because, for instance, they leave their stomata open for as long as possible or have embolism-prone xylem or shallow roots. “Many of the species that you find there, they can cope with five months without rain,” says University of Minnesota forest ecologist Jennifer Powers. “But when you give them seven months without rain, it doesn’t matter if it rains two meters during the rainy season.”
One of the most surprising episodes played out in 2011 when, after a severe dry period and a series of heat waves, scientists noticed many trees shedding their leaves in the Northern Jarrah Forest in southwestern Australia. The eucalyptus trees there — predominantly the jarrah tree Eucalyptus marginata — eagerly resprout after wildfires and endure up to seven months of drought each year, slurping groundwater through roots that can extend 50 meters (more than 150 feet) down. Conventional wisdom was that this forest was bombproof, says ecologist Joe Fontaine of Murdoch University in Perth. But that year, many trees lost their crowns entirely and resprouted from the trunk, only to give up on the trunk and resprout from the base before ultimately dying — “almost like the aftershocks of an earthquake,” Fontaine recalls.
Though many experts were startled, perhaps they shouldn’t have been, says plant physiologist Tim Brodribb of the University of Tasmania. These fast-growing trees are late to close their stomata, their xylem shunts water quickly but is prone to embolizing, and they drink water voraciously until it runs out, as it probably did in the Jarrah forest due to years of declining rainfall. “Everyone thinks of eucalypts as being really tough, but in fact, they’re just really sleazy pioneers,” Brodribb says.
The fundamental dilemma is that trees often have to make trade-offs: They can spend their carbon on rapid growth or on building a strong hydraulic system, but they usually cannot afford to do both. Eucalypts opted for the former — allowing them to overwhelmingly dominate in the majority of Australian forests but causing them to die in droves during droughts. By contrast, Callitris, a conifer genus in the cypress family that features in other forests of that continent, has chosen to invest in its hydraulic system, sacrificing an ability to regrow rapidly and compete in a fire-prone landscape.
Callitris xylem is so robust that when Brodribb had a colleague spin a piece of it in a centrifuge to find out when it would develop dangerous embolism levels, they had to spin it at such high speeds that the centrifuge broke instead.
The trouble is many tree species have opted for a risky strategy that may be too risky in today’s warming world. In a 2012 study, Brodribb and his collaborators amassed information for 226 tree species from 81 sites around the globe. They collected data on the water potentials at which hazardous embolisms occur and the average water potentials that the species are normally at in the wild. They found that 70 percent of species hovered very close to this dangerous threshold — because, for instance, they were slow to close their stomata, had a weak xylem, or had to work harder to rehydrate due to shallow root systems.
Remarkably, this was true across all forest types examined. From dry forests to temperate forests to tropical forests, many tree species accept being on the brink of hydraulic failure because it helps them to outcompete other trees.
But while that strategy worked well before human-caused climate change, the more extreme droughts brought by today’s climbing temperatures are too much for trees to handle. “Drought looks different in the Amazon than it does in Arizona,” says landscape and forest ecologist Craig Allen of the University of New Mexico — but in each region, trees are adapted to local conditions that a changing climate has upended, so they’re bumping against thresholds of what they can tolerate.
Warmer temperatures don’t just push trees to the hydraulic edge. The droughts they bring supercharge other stressors, such as fire: A spring drought likely contributed to 2023’s record-breaking wildfire season in Canada, for example. Even on the fringes of the Amazon rainforest, drought is making it easier for people to burn areas for plantations and for the fires to spread farther — although the moist interior of the forest still seems relatively resilient, says University of Birmingham ecologist Adriane Esquivel-Muelbert.
Worldwide, some studies estimate that fires now burn about twice the amount of forest as they did in 2001. In 2021 — a particularly bad year — burns consumed 9.3 million hectares, a Portugal-size area.
A perfect storm
For most trees that die during droughts, diseases or insects like bark beetles are usually the fatal blow. It’s true for European spruces as well as exceptionally hardy species like bristlecone pines and the giant sequoias of the Sierra Nevada in the United States. Between 2014 and 2020, forest ecologist Nathan Stephenson saw 33 giant sequoias die; he suspects that a combination of fire and drought interrupted water flow to the trees’ crowns, leaving them unable to push out defensive resins against beetles. A beetle species that’s never been known to kill sequoias then invaded from the crown downward. “What finally took them out was a native bark beetle that was too weak to kill them under normal conditions but could finally kill them under extreme conditions,” says Stephenson, a scientist emeritus at the US Geological Survey.
This constellation of crises is also rife in the mountainous regions of northern New Mexico, where Allen has been documenting the impacts of a regional megadrought that began in 2000. Regular droughts had struck the region before. But this time, a century of fire suppression and a massive buildup of dense vegetation during a previous wet period — along with warmer temperatures that set in with the drought — caused all hell to break loose, Allen says.
Bark beetle populations exploded in the warmth, with more larvae surviving the winter and squeezing more generations into a season. Between 2002 and 2004, they devoured more than 1 million hectares each of piñon pine (Pinus edulis) and Ponderosa pine (Pinus ponderosa) in the Southwest. The trees likely couldn’t muster enough carbon or water to produce the resin that would normally protect them. Fires feasted on the dense vegetation.
Over and over, thick, vigorous conifer stands that Allen had known for decades transformed into shrubs and grasslands, with so few trees in places that today he can see the next mountain ranges, dozens of miles away. “A lot of my favorite trees and forests from the ’80s and ’90s are not alive anymore,” he says. “I know ecosystems are dynamic — I know that intellectually. But it’s one thing to know, and it’s another to live through the magnitude of transformations that have gone on in this landscape.”
An uncertain future
Until recently, many scientists believed that, overall, rising carbon emissions would be good news for forests for the simple reason that plants need carbon dioxide to grow. If there’s more of it in the air, they get more carbon dioxide for each molecule of water they lose — allowing them to build tissues faster and use water more efficiently.
That’s why the first computational models of vegetation growth under climate change showed a widespread greening of the planet — and, indeed, recent satellite studies show that there was an expansion in worldwide vegetation during the 1980s and 1990s.
But it’s increasingly evident that these benefits may be outweighed by the warming effects of carbon emissions. According to one 2019 study, global greening stopped more than 20 years ago, and vegetation has been declining since, all because of the drought-amplifying effects of warming.
As the atmosphere gets warmer, it gets thirstier, and this relationship is exponential, such that for every 1 degree Celsius of warming, the atmosphere can hold 7 percent more water. The bonus carbon dioxide is of little use to trees that close their stomata to protect themselves from losing water or dying outright due to supercharged droughts.
Some studies suggest that trees cannot create wood under too much drought stress, even when they’re photosynthesizing; instead, they may expel the carbon out through their roots. Rather than feasting on carbon dioxide and helping to counteract climate change, forests might largely suffer from the elevated emissions. And when they rot or burn, they will spew carbon back into the air, amplifying global warming.
The noxious trio of drought, insects, and fire “could be the difference between flipping the land surface from a carbon sink to a carbon source,” says Anna Trugman, a global change ecologist at the University of California, Santa Barbara.
Weak knees and a shaky future
The scientists in Hölstein are well aware of these questions. Before I leave the site, Peters and I climb up a series of ladders inside the crane scaffold and watch as Steger, in the gondola, uses a device to measure the conifers’ leaves to determine their rate of photosynthesis.
From this height — at which Peters clearly feels quite comfortable but where my knees become decidedly wobbly — we get a good view of the plastic rain roofs, propped like greenhouses above the forest floor. Peters says that if more extreme droughts occur, they’ll be reluctant to use the roofs out of fear that some trees will die outright.
Right now, scientists don’t know how many trees will succumb to drought. Indeed, despite the dramatic stories of ravaged forests, some researchers remain reluctant to say for sure that drought-driven die-offs are a worsening trend simply because there isn’t enough data on tree losses globally to know.
Since 2010, Allen and others have been compiling a database of reported forest die-offs due to heat and drought from around the world. The database started with 88 episodes and now lists more than 1,300. But this isn’t a comprehensive picture of what’s happening in the world’s forests, Hartmann says; some of the largest forests on Earth — the boreal and tropical woodlands — are under-studied.
It’s also very hard to predict how bad die-offs could get in the future because the mathematical simulations used to predict vegetation responses are based on outdated assumptions about how trees respond to drought, Hartmann says. When he and his colleagues recently used a “dynamic global vegetation model” to see if it could predict any of the stunning die-off events in places like Germany, Australia, and the southwestern US, it failed to predict the die-off history of even one of them reliably.
Most vegetation models don’t incorporate the hydraulic processes that Kahmen and others are now learning are critical to a tree’s survival, as they’re complex and still not fully understood. Instead, they largely focus on processes that occur in the leaves, like photosynthesis. When trees die in these models, they usually do so by starving when their stomata are closed, and they can’t get enough carbon — and many researchers, including Hartmann, think this is unlikely to be the sole cause of death during drought.
Trugman says that modelers are slowly catching on and have begun to incorporate metrics that reflect the stress in a tree’s hydraulics, like how quickly a tree loses its capacity to transport water. She points to one model described in a 2018 study that correctly predicted when individual trees in a research garden would reach hydraulic failure. However, it has proved challenging to do this over larger spatial scales. One big unknown is the vast, dynamic world of groundwater, how much is available to forests, and which tree types have the roots capable of reaching it.
It will take years, Trugman adds, before hydraulic processes are understood and modeled well enough to be properly represented in die-off predictions for the IPCC’s big climate change projections, which are intended to guide governmental policies.
Another mystery is the scale of compounding stressors like wildfires and insect infestations in the future; the latter isn’t reflected in any of the IPCC models either. There are copious species of bark beetle, and researchers know little about how each will respond to environmental change. “We’re going to see new interactions that we haven’t seen before,” Stephenson says. “To be able to anticipate those ahead of time is in some cases just going to be impossible.”
This article originally appeared in Knowable Magazine, an independent journalistic endeavor from Annual Reviews. Sign up for the newsletter.
This article was originally published on