Superbugs' own biology may be the ultimate weapon against them — study
An approach like this may be a game-changer in the fight to eliminate dangerous antibiotic-resistant bacteria.
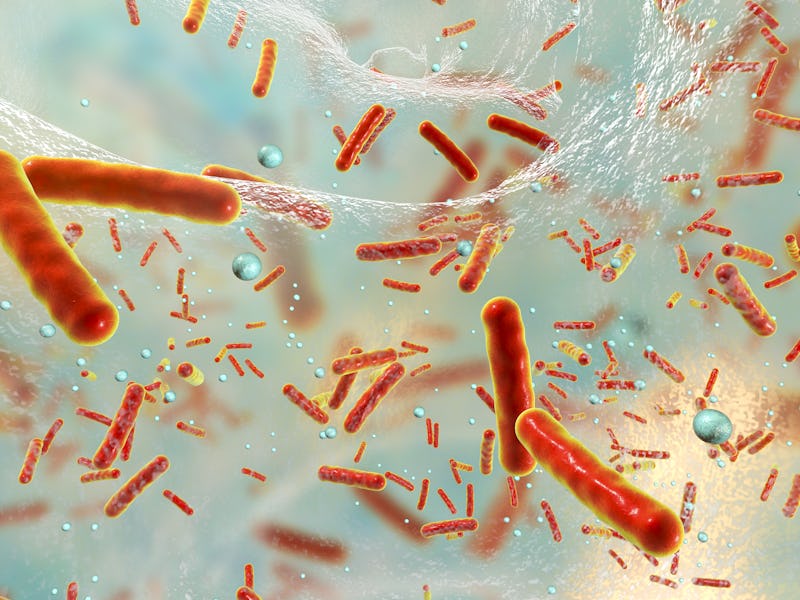
Scientists discovered and began using antibiotics to treat polio in 1928. A game-changer for medicine, the drugs have since evolved to treat a plethora of illnesses, and antibiotic-resistant bacteria have been evolving alongside, just waiting to take back control.
And in many ways, they already have.
Antibiotic-resistant superbugs are a problem in hospitals worldwide. They threaten the effectiveness of life-saving antibiotics, making people increasingly vulnerable to once deadly bacteria, like Staphylococcus aureus (the cause of staph infections).
But a new study published Wednesday in the journal Nature suggests the effort to safeguard the future of antibiotics may be coming close to fruition. Synthetic chemists have discovered a new way to create antibiotics that use their bacterial nemeses' own tricks against them.
In the study, researchers test their newly synthesized antibiotics on mice, showing that these novel drugs significantly inhibited resistant bacteria's growth.
The reason why appears to be to do with the bacteria's own biology.
Thus far, the fight against superbugs has involved trying to create new antibiotic molecules from scratch that can overcome antibiotic resistance. But this process is neither cheap nor simple, and would be difficult to scale to a global level.
Instead, the team of scientists behind the new study have tweaked an existing antibiotic molecule using Lego-like, molecular building blocks to draw out un-utilized traits. This small change allows the molecule to naturally avoid an "ancient antibiotic mechanism." The authors of a companion report write that this approach could be a powerfully adaptive tool.
"This Lego-like synthetic strategy allows molecular building blocks to be readily swapped, thus providing access to many targeted derivatives," write the authors. "Such modularity is a powerful enabler of creativity: because many variants can be rapidly assembled and tested, researchers can make almost any target that they want."
By changing the location of a single bump on this antibiotic, researchers were able to block bacteria's resistance to it.
Multi-pronged attack — The team focused in on a member of the streptogramin antibiotic family, Virginiamycin M2 (VM2), which uses a two-pronged attack to adhere to and inhibit the growth of bacteria.
Bacteria that have developed resistance to this antibiotic do so by binding to it and forcing it to grow a small little bump that prevents it from easily sliding into place in the bacteria's ribosomes. Without this first prong successfully landing, the second one becomes ineffective as well, and so the bacteria proliferate.
To overcome this problem, the researchers decided to reverse-engineer the antibiotic molecule to essentially use the same tactic against the bacteria.
When done by the bacteria, enzymes deactivate and reshuffle different parts of the molecules' chemical make-up to cause its bump. In their study, the researchers found a molecule that mirrors this same bump mechanism and used it to place the bump at the other end of the antibiotic. The switch means the bacteria can't bind with the antibiotic, thus stopping them from switching the molecules' chemical make-up. As a result, the antibiotic was able to carry-out its two-pronged attack on the bacteria's ribosome without a problem.
To test how effective this strategy was in a real, living organism, the researchers looked at how their solution would fair against streptogramin-resistant strains of Staphylococcus aureus in mice. They found the re-imagined antibiotics were effective at lowering the overall bacterial load in these mice.
What's next — The study is in mice and the findings can't be translated to humans. The results are promising, but the researchers caution their strategy isn't necessarily a permanent solution to antibiotic resistance. Rather, it could help extend the usefulness of certain antibiotics while researchers continue to design new ones.
"Although the emergence of other resistance mechanisms is inevitable, this approach may permit chemical adaptations to extend the clinical longevity of the streptogramin class," write the authors.
The next step will be to conduct more animal and, eventually, human trials to determine the clinical potential of such an approach. But the companion report authors suggest the innovative use of chemistry in this study will move the needle forward for the field nonetheless.
"More broadly, this story will embolden the pursuit of modular, complex small molecules as engines for pushing the frontiers of chemical biology and drug discovery," they write.
Abstract: Natural products serve as chemical blueprints for most antibiotics in clinical use. The evolutionary process by which these molecules arise is inherently accompanied by the co-evolution of resistance mechanisms that shorten the clinical lifetime of any given class of antibiotics. Virginiamycin acetyltransferase (Vat) enzymes are resistance proteins that provide protection against streptogramins, potent antibiotics against Gram-positive bacteria that inhibit the bacterial ribosome. Owing to the challenge of selectively modifying the chemically complex, 23-membered macrocyclic scaffold of group A streptogramins, analogues that overcome the resistance conferred by Vat enzymes have not been previously developed. Here we report the design, synthesis, and antibacterial evaluation of group A streptogramin antibiotics with extensive structural variability. Using cryo-electron microscopy and forcefield-based refinement, we characterize the binding of eight analogues to the bacterial ribosome at high resolution, revealing binding interactions that extend into the peptidyl tRNA-binding site and towards synergistic binders that occupy the nascent peptide exit tunnel. One of these analogues has excellent activity against several streptogramin-resistant strains of Staphylococcus aureus, exhibits decreased rates of acetylation in vitro, and is effective at lowering bacterial load in a mouse model of infection. Our results demonstrate that the combination of rational design and modular chemical synthesis can revitalize classes of antibiotics that are limited by naturally arising resistance mechanisms.