Scientists may be on the verge of finally unraveling mystery of antimatter
"The sheer existence of humans shows that our Standard Model is wrong."
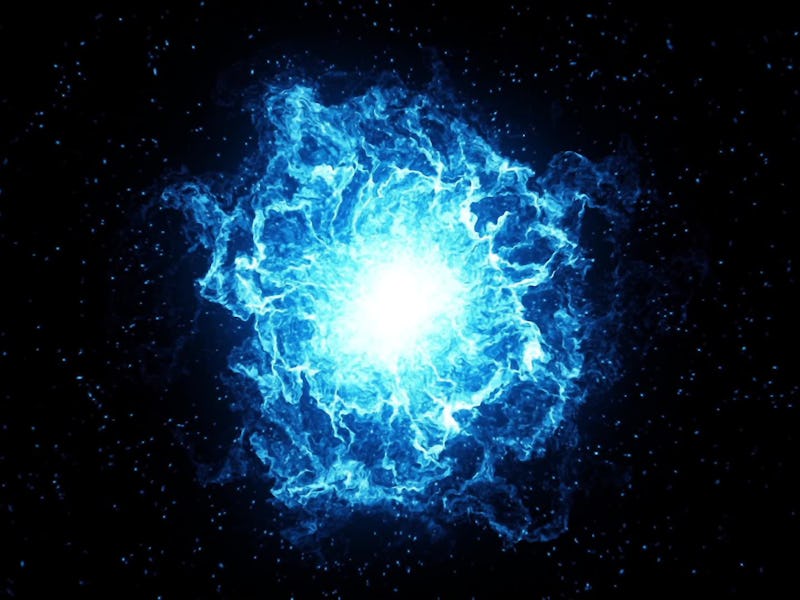
Scientists are a step closer to understanding one of the fundamental properties of antimatter — and it is as weird as they expected.
The new research zooms in on antihydrogen is the mysterious equivalent of hydrogen, made of antimatter. As opposed to 'ordinary' matter, which is composed of particles like protons, antimatter is composed of antiparticles, like antiprotons — a kind of opposite-but-equal, funhouse mirror-image of matter.
In theory, a molecule of antihydrogen would have the same but opposite properties as a molecule of hydrogen — and if the two met, the collision would result in their mutual destruction. The two opposites literally cancel each other out.
The new milestone measurements reported in the journal Nature on Wednesday show that antihydrogen performs one of the same shifts in energy that hydrogen does. These details — which had only been theorized before — may help determine whether the two opposite particles are symmetrical or not. And that could help scientists understand whether matter and antimatter are truly parallel.
The findings have critical implications for our understanding of the Standard Model, the physics theory which science uses to explain the universe and why it is the way it is.
“You know, 30 years ago or so when people started dreaming about this, they kind of drew up a list of things that you better check to convince yourself that matter and antimatter are complete parallels,” Jeffrey Hangst, lead researcher at the ALPHA Collaboration at CERN and a scientist on this project, tells Inverse.
“This is number two or three on the list of the boxes you need to check. My experiment is the only one in the world that is able to do that as of today.”
Why does antimatter matter?
To understand why the findings are so important to our understanding of nature, the universe, and, really, everything as we know it, let us zoom out.
Everything in the universe is made up of matter. In turn, matter is built up by particles, such as neutrons, protons, electrons, and more. According to the current laws of physics, when the Big Bang created all of these particles, it also created equivalent, opposite particles — antineutrons, antiprotons, antielectrons, and so on — this is known as antimatter.
Since matter and antimatter are characterized by opposite properties, they will annihilate each other when they meet. Think of it like the sum 1 + -1 = 0. Poof.
Antimatter was first proposed in 1928, by British physicist Paul Dirac. In 1932, another scientist, Carl Anderson, discovered the positron, or antielectron, helping to confirm Dirac's theory — at least in part.
Thankfully, antimatter does not naturally occur on Earth. To observe it, researchers need to create it using complicated atomic reactions. Particle accelerators, like the one at CERN, for example, create tiny numbers of antiparticles when particle beams collide. Because it is so scarce on Earth, antimatter is extremely hard to study, measure, and, therefore, to understand — as a result, scientists know very little about it, almost a century after it was first proposed to exist.
“Looking at the structure of an atom is something we understand really, really well, and we know how to do it very precisely on matter,” Hangst says.
“So that's the attraction here — to do really careful tests to see if there is any difference between the physics of matter and antimatter.”
Antimatter is critical to our understanding of the universe. It could hold the key to how the world exists in the way that it does — ultimately, it may help answer questions science doesn’t even know how to ask yet.
"The sheer existence of humans shows that our Standard Model is wrong."
“We have this beautiful Standard Model which is very nice and fine. And it describes almost everything that we can see,” Randolf Pohl, a physicist at Johannes Gutenberg-Universität Mainz tells Inverse. “But we know that it must be incomplete, so it must be wrong in some sense because the Standard Model cannot explain why we are here.”
Pohl was not involved in the new study.
If the Big Bang really made equal amounts of matter and antimatter — as the Standard Model dictates — then they would simply have annihilated each other. There would be nothing left to form the galaxies, stars, and planets that make up the universe — or indeed, humans.
"The sheer existence of humans shows that our Standard Model is wrong," Pohl says.
“With only the Standard Model, we should not exist. There must be some difference between matter and antimatter that we don't know yet about. And so studying and comparing particulates and the antiparticles is one way to try and find some problem of the Standard Model to make it a more complete theory.”
This new study puts us firmly on the path to understanding what exactly we are missing, Pohl says.
“I think this is a very important step towards very precise tests of the Standard Model, because, hydrogen is, for physics, something like the Rosetta stone,” Pohl says.
What do we know about antihydrogen?
We know a lot about hydrogen. It is the most simple and most abundant particle in the universe. But we know very little about antihydrogen.
“The so-called Standard Model of how the world is screwed together says that this antihydrogen, this exotic thing we make in the laboratory, should have the same exact properties as hydrogen,” Hangst says.
Hangst and his team prove that antihydrogen and hydrogen share at least one fundamental property: Lamb shift. The Lamb shift describes a shift in the difference in energy levels within the fine structure of the hydrogen spectrum.
Here is how Hangst explains it: First, the scientists produce and trap antihydrogen.
"It's kind of held in a magnetic bottle in an ultra high vacuum," he says.
Then, they excite the antihydrogen with a laser, getting it to a transition point and then annihilating it by bringing it into contact with hydrogen.
They then measure the two different transitions in antihydrogen from its base state to the excited state. The difference between these two energy levels is a single quantity, called the "fine structure splitting," Hangst says. From there, the scientists can derive the Lamb shift. The Lamb shift is the difference between the two levels.
It is just one property — but confirming that both antihydrogen and hydrogen exhibit it is fundamental to our understanding of how the universe works.
“This is really fundamental research at the limit of our understanding.”
“We are kind of tracing the history [of hydrogen] in a historically parallel way — on the antihydrogen spectrum — to see if all these things are the same,” Hangst says.
“It's not the first measurement on antihydrogen, but it's the first one of this character.”
The more scientists learn about hydrogen, the more they will need to keep learning about antihydrogen. It is like chasing a moving target, Hangst says.
Ten years ago, observing antihydrogen was not even possible.
“This is really fundamental research at the limit of our understanding,” Hangst says.
“We are looking at the most basic questions that you can ask about what are the symmetries of the universe. How is it actually? What is the structure of space and time?”
Abstract: At the historic Shelter Island Conference on the Foundations of Quantum Mechanics in 1947, Willis Lamb reported an unexpected feature in the fne structure of atomic hydrogen: a separation of the 2S1/2 and 2P1/2 states1 . The observation of this separation, now known as the Lamb shift, marked an important event in the evolution of modern physics, inspiring others to develop the theory of quantum electrodynamics2–5. Quantum electrodynamics also describes antimatter, but it has only recently become possible to synthesize and trap atomic antimatter to probe its structure. Mirroring the historical development of quantum atomic physics in the twentieth century, modern measurements on anti-atoms represent a unique approach for testing quantum electrodynamics and the foundational symmetries of the standard model. Here we report measurements of the fne structure in the n = 2 states of antihydrogen, the antimatter counterpart of the hydrogen atom. Using optical excitation of the 1S–2P Lyman-α transitions in antihydrogen6 , we determine their frequencies in a magnetic feld of 1 tesla to a precision of 16 parts per billion. Assuming the standard Zeeman and hyperfne interactions, we infer the zero-feld fne-structure splitting (2P1/2–2P3/2) in antihydrogen. The resulting value is consistent with the predictions of quantum electrodynamics to a precision of 2 per cent. Using our previously measured value of the 1S–2S transition frequency6,7, we fnd that the classic Lamb shift in antihydrogen (2S1/2–2P1/2 splitting at zero feld) is consistent with theory at a level of 11 per cent. Our observations represent an important step towards precision measurements of the fne structure and the Lamb shift in the antihydrogen spectrum as tests of the charge– parity–time symmetry8 and towards the determination of other fundamental quantities, such as the antiproton charge radius9,10, in this antimatter system.