Astronomers Capture Space-Squishing Echoes of Merging Supermassive Black Holes
Several teams of scientists from around the world all report detecting extremely low-frequency waves in spacetime, caused by merging supermassive black holes.
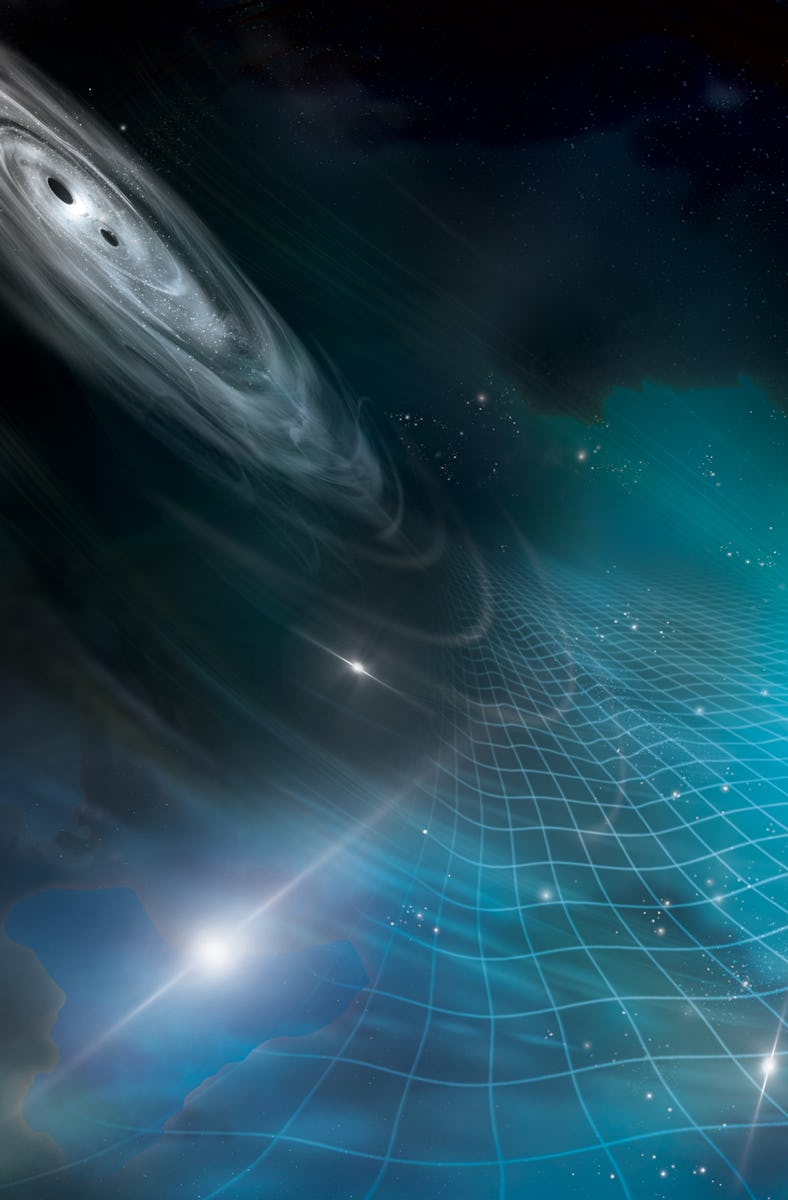
There’s a monster lurking at the center of every galaxy, millions of miles wide and millions of times more massive than our Sun: a supermassive black hole. When two of these cosmic leviathans meet, they fall into a million-year death spiral that ends in a dramatic merger.
The motion of these cosmic leviathans creates long, low-frequency waves in the fabric of spacetime, called nanohertz gravitational waves. Astronomers just measured these extremely low-frequency gravitational waves for the first time — by turning a whole quadrant of our galaxy into a giant astronomical instrument.
“We’re using a gravitational wave detector the size of the galaxy that’s made out of exotic stars, which just blows my mind,” says the National Radio Astronomy Observatory’s Scott Ransom in a statement.
The U.S.-based NANOGrav collaboration published their findings in a series of papers in The Astrophysical Journal Letters. Other research groups based in Australia, China, Europe, and India made the same discovery independently and are publishing their own papers at the same time. You can read the papers here, here, here, and here.
Ripples in Space
When galaxies collide and merge, their supermassive black holes eventually merge as well, and that sends nanohertz gravitational waves rippling through our galaxy.
Einstein’s theory of general relativity predicted in 1916 that when really enormous objects move around, they should make waves in the very fabric of reality, called spacetime. A century later, in 2015, the Laser Interferometer Gravitational-wave Observatory (LIGO) proved that with its first detection of a gravitational wave. In this case, the waves were kicked up by a merger between two ordinary black holes, each about 30 times the mass of our Sun.
What NANOGrav recently observed was happening on a scale millions of times larger than that. LIGO used 2.5 mile-long laser beams to measure how waves in spacetime stretched our planet as they passed through. But our entire planet is too small to measure the gravitational waves stirred up when supermassive black holes collide. Their waves are huge (billions or trillions of miles long) and slow (with years or decades between when one wave passes and the next arrives).
NANOGrav, an alliance of about 190 astronomers and astrophysicists, along with other groups of researchers around the world, found the instrument they needed in the shrieking cores of dead stars scattered around the galaxy.
This image of our Milky Way Galaxy shows the locations of the pulsars that make up NANOGrav’s pulsar timing away.
When some massive stars explode into supernovae, they leave behind a densely packed ball of neutrons called a neutron star. Some neutron stars, called millisecond pulsars, blast beams of radio waves out into space, like the beams of light from a lighthouse rotating hundreds of times a second — so what astronomers see from Earth is a pulsing radio signal, as regular as cosmic clockwork.
As gravitational waves roll slowly past a pulsar, they disrupt the dead star’s rotation; picture a buoy at sea being nudged by passing waves, so its light seems to flicker. The wave also stretches or squishes the space in between the pulsar and Earth, so the radio waves arrive either earlier or later than normal.
NANOGrav’s researchers and their international colleagues spent the last 15 years watching 68 pulsars with some of the world’s most sensitive radio telescopes, like Arecibo (RIP), the Green Bank Telescope, and the Very Large Array.
“Pulsars are actually very faint radio sources, so we require thousands of hours a year on the world’s largest telescopes to carry out this experiment,” says NANOGrav PFC co-director Maura McLaughlin, of West Virginia University, in a statement.
The result is what the researchers call a pulsar timing array. By watching the effect on all of those pulsars, the astronomers were able to see the effects of gravitational waves moving across the galaxy like huge, slow rollers moving across the ocean.
“The changes produced in a pulsar are related to the changes produced in another pulsar. Finding this correlation pattern is how we know that what we are seeing is caused by gravitational waves,” says Sarah Vigeland of the University of Wisconsin in Milwaukee tells Inverse.
If LIGO’s detection of colliding stellar-mass black holes was the equivalent of ripples from a stone tossed into a pond, then nanohertz gravitational waves are constant, the way the ocean is always in motion here on Earth. What NANOGrav and the other pulsar timing arrays measured was the overlapping motion of gravitational waves from the estimated hundreds of thousands of black hole binaries in the universe, formed by hundreds of thousands of mergers between galaxies.
“It doesn’t come from a single source,” says Vigeland. “Instead, it’s built up from the gravitational waves emitted by many sources from throughout the universe. Each source emits a particular signal, like a single note, and then the combination of all of them produces a sort of ‘hum’ of gravitational waves.”
This illustration demonstrates how supermassive black hole mergers create gravitational waves, and how pulsar timing arrays detect them.
A Cosmic Orchestra
Eventually, astronomers hope to find the individual notes in that ‘hum,’ or rather, the individual waves in the constant background undulation of spacetime — tracing them back to the pairs of merging supermassive black holes that spawned them. Doing so could help shed more light on how the large, intricately-structured spiral galaxies of the modern universe evolved from smaller, simpler galaxies over billions of years, and how they’re still evolving today.
Meanwhile, one of the next steps for the researchers will be to compare and combine the data from all of the pulsar timing arrays around the world. The four teams who recently published their work communicated extensively, but they all gathered and analyzed their own data.
“The fact that they are broadly consistent is a good sign,” says Vigeland.
But for now, the gravitational wave background has helped solve a sticky problem with supermassive black holes, something astrophysicists call the “final parsec problem.”
When two galaxies merge, their respective supermassive black holes eventually, in theory, merge into a new, even more massive black hole. But the physics of two of the densest, most mysterious objects in the universe actually colliding and merging is, well, complicated and difficult.
“At one point, scientists were concerned that supermassive black holes in binaries would orbit each other forever, never coming close enough together to generate a signal like this,” says UC Berkeley’s Luke Kelley in a statement.
Once a pair of supermassive black holes get close enough together to stir up nanohertz gravitational waves, there’s no hope for them — they’re doomed to collide and merge within a few million years. In other words, the gravitational wave background proves that supermassive black holes really can pull off a merger.
This article was originally published on