The Large Hadron Collider restarts next week — here's what it's hunting for
Here's what you need to know.
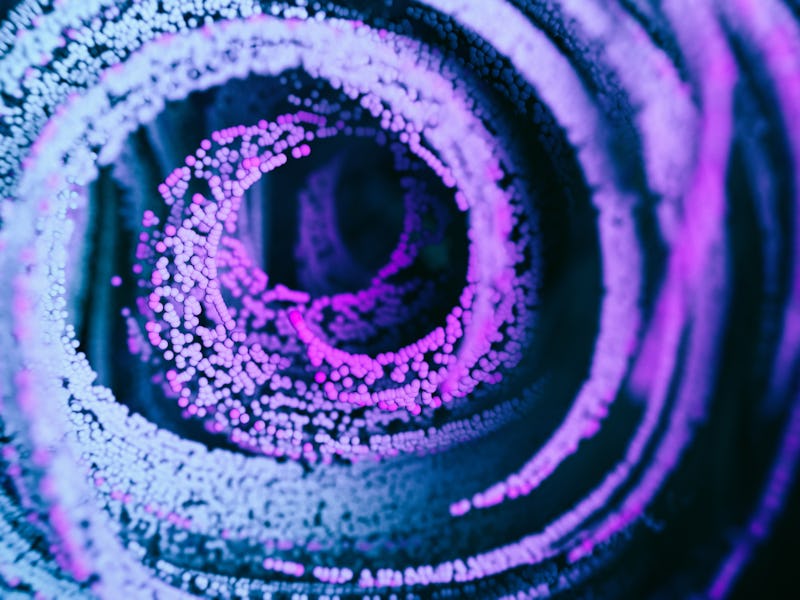
On July 5, underneath the suburbs of Geneva, Switzerland, the world’s largest particle collider will fire up and start collecting data again. And what they might find has the potential to blow particle physics wide open.
After nearly four years of shutdown, extended by Covid-induced delays, the Large Hadron Collider (LHC) is about to kick off its third round of experiments: called, succinctly, Run 3. CERN will commemorate the launch with a livestream at 10:00 AM, Eastern time.
Physicists have high hopes for Run 3. They hope to unlock new particles and new mechanisms that they’ve never been able to see. Recent physics research has unveiled a possible fifth force and challenges to the Standard Model of physics. Run 3 could
What does the Large Hadron Collider do?
The LHC is a particle collider. The name accurately depicts what LHC does: it smashes particles — usually protons, but it can also collide larger particles that physicists call “heavy ions.” Typically, that means ions of lead, the heaviest non-radioactive element.
To do this, the LHC first shoots two beams of particles into its ring, traveling in opposite directions. They spiral round and round, accelerated and guided by high-powered electromagnets, until they reach very near the speed of light. Then, after they’ve come up to speed, they collide head-on.
The ALICE Experiment at CERN, which deals with large ions.
Those collisions cause the innards of the speeding particles — the tinier particles that work as their building blocks — to fling loose. Some crash into each other. And in the high-energy, high-temperature, high-extreme conditions within a collision, all sorts of weird particles can pop out of the woodwork.
Scientists study the detritus that’s left behind. Their highly sophisticated detectors can pick through the debris and find the trails, traces, and fingerprints all those particles leave behind.
Large Hadron Collider purpose
Smashing particles together sounds like a crude way to learn about them: a bit like smashing complex electronic devices together and hoping to learn how they work from the mangled components that are left behind. But it’s the best way that physicists have to look into the quantum world, at scales millions of times smaller than even atoms.
But in those collisions, many of these particles are phantoms, barely interacting with the world or lasting fractions of a second. Typically, they’d go unseen, even if you look at them with very powerful detectors. But scientists can find those particles' telltale signatures in the high-energy soup that emerges for an instant inside a particle collider like LHC.
The upgrades to the LHC over the shutdown have boosted its energy, giving it even more power to unveil this subatomic world.
Large Hadron Collider size
The LHC is a behemoth. It’s housed in a circular tunnel, 27 kilometers (17 miles) in circumference and 4 meters (13 feet) wide, buried several stories underground. From CERN’s headquarters in the Geneva suburbs, this tunnel streaks under the towering Jura Mountains, along the undulating French-Swiss border, and comes back again.
LHC is so large because, with more circumference for a particle beam to accelerate through, particles can get ever closer to the speed of light and, therefore, carry higher energies. With higher energies, physicists can see more particles when beams collide.
As massive as the LHC is, scientists aren’t afraid to dream even bigger. If some scientists have their way, LHC will have a future successor — a so-called Future Circular Collider — that has nearly four times the circumference.
The computing grid for the LHC, responsible for processing the petabytes of information produced by the experiments.
Large Hadron Collider discoveries
Perhaps LHC’s highest-profile discovery so far has been the Higgs boson. According to particle physics, this ghostly particle is a product of something known as the Higgs field, which gives mass to certain particles, the W and Z bosons. These particles guide the weak nuclear force that governs some forms of radioactivity.
By finding the Higgs boson, particle physicists could confirm that much of their theory about how the universe works at tiny scales was correct. But the Higgs boson is highly unstable, and observing it has to contend with the fact that it will break apart almost instantaneously.
The Higgs boson was first proposed as early as the 1960s, and since then, scientists sought it for decades until it was finally discovered in 2012 at the LHC. In fact, the quest for the Higgs boson was one reason that LHC was built in the first place. Earlier particle colliders didn’t have the energy needed to find it.
Even though scientists have found a Higgs boson, they don’t understand its properties well. Doing that is on their wishlist.
What are the new Large Hadron Collider experiments?
There aren’t *new* experiments per se — but they build on existing ones in search of unknown physics.
LHC isn’t just one big experiment. It actually hosts multiple experiments. Each one is looking for different particles or examining different physics. Each one has its own detector located somewhere along the accelerator’s loop. Each one is supported by as many as hundreds of scientists all over the world.
There are four big ones. ATLAS and CMS are “general-purpose” experiments, looking at a wide range of particles that pass through their respective detectors’ scrutiny. These two experiments found the Higgs boson.
Illustrations of the CMS and ATLAS detectors.
ALICE hopes to study a quirky phase of matter known as “quark-gluon plasma,” where atoms literally melt away into a superhot soup. Cosmologists believe that quark-gluon plasma dominated the universe for a brief moment, early in its history.
LHCb (short for “LHC beauty”) aims to examine one particular particle, called the beauty quark. Scientists think the beauty quark can teach them more about the differences between matter and its opposite-charge, destructive twin: antimatter. When matter and antimatter touch, they annihilate each other. The Big Bang ought to have created matter and antimatter in equal amounts, but it seems to have created excess matter— the matter that surrounds us. This imbalance has no explanation.
There are several smaller experiments, many of them looking at other specific particles or other elements of physics.
What do CERN scientists hope to find?
For decades now, particle physics has lived and died by the so-called Standard Model. It’s a schema that neatly lays out the fundamental particles of the universe — 17 of them — and how they interact with each other. It governs three of the universe’s four fundamental forces: the strong nuclear force, which holds particles together inside the nucleus of an atom; the weak nuclear force, which guides some forms of radioactivity; and electromagnetism.
For decades, particle physics seems to have almost always obeyed the Standard Model’s predictions — almost.
Particle physicists are increasingly of the mind that the Standard Model isn’t all there is. There are a few curiosities that the Standard Model doesn’t satisfy. For instance, the model does not answer the fourth fundamental force: gravity. Nor has it (so far) given a satisfactory culprit for dark matter, which is more than five times greater in abundance than “normal” matter.
Some of these unanswered questions have made scientists suspect that there’s a fifth fundamental force lurking somewhere out there. One idea is that this fifth force is somehow linked to dark energy, a mysterious form of energy that seems to be causing the universe to accelerate.
Some experiments have hinted at particles beyond the Standard Model, perhaps the bearers of physics beyond scientists’ current understanding.
Recently, scientists poring over old data from a different particle accelerator at Fermilab in suburban Chicago found that one particle, the W boson, has a higher mass than expected. It sounds minor, but it’s a serious breach of the Standard Model. Physicists hope that LHC can help them test this.