Animal brains use complex calculations to evade getting eaten
Scientists are beginning to unravel the complex circuitry behind the split-second decision to beat a hasty retreat.
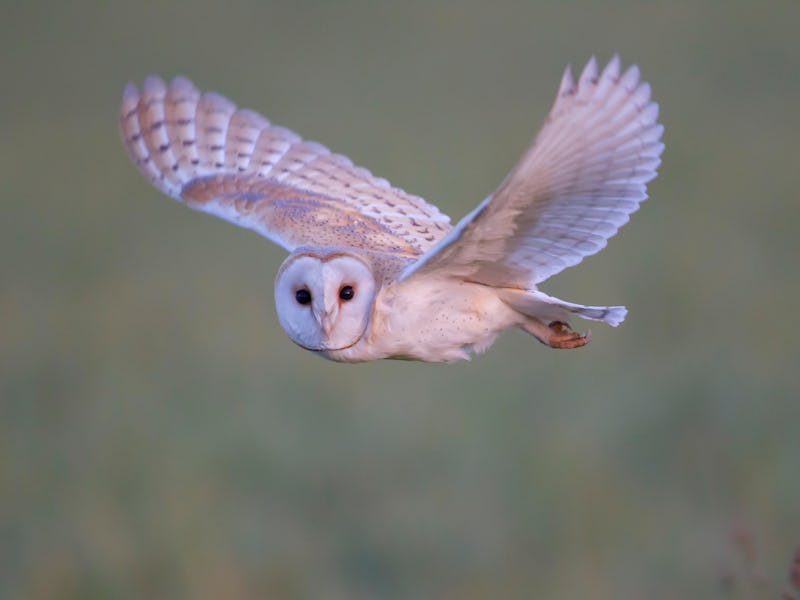
Survival of the fittest often means survival of the fastest. But the fastest doesn’t necessarily mean the fastest moving. It might mean the fastest thinking. When faced with the approach of a powerful predator, for instance, a quick brain can be just as important as quick feet.
After all, it is the brain that tells the feet what to do — when to move, in what direction, how fast, and for how long. And various additional mental acrobatics are needed to evade an attacker and avoid being eaten. A would-be meal’s brain must decide whether to run or freeze, outrun or outwit, whether to keep going or find a place to hide. It also helps if the brain remembers where the best hiding spots are and recalls past encounters with similar predators.
All in all, a complex network of brain circuitry must be engaged and neural commands executed efficiently to avert a predatory threat. And scientists have spent a lot of mental effort themselves trying to figure out how the brains of prey enact their successful escape strategies. Studies in animals as diverse as mice and crabs, fruit flies, and cockroaches are discovering the complex neural activity — in both the primitive parts of the brain and in more cognitively advanced regions — that underlies the physical behavior guiding escape from danger and the search for safety. Lessons learned from such studies might not only illuminate the neurobiology of escape but also provide insights into how evolution has shaped other brain-controlled behaviors.
This research “highlights an aspect of neuroscience that is really gaining traction these days,” says Gina G. Turrigiano of Brandeis University, past president of the Society for Neuroscience. “And that is the idea of using ethological behaviors — behaviors that really matter for the biology of the animal that’s being studied — to unravel brain function.”
Think fast
Escape behavior offers useful insight into the brain’s inner workings because it engages nervous system networks that originated in the early days of evolution. “From the moment there was life, there were species predating on each other and therefore strong evolutionary pressure for evolving behaviors to avoid predators,” says neuroscientist Tiago Branco of University College London.
Not all such behaviors involve running away, Branco notes. Rather than running, you might jump or swim. Or you might freeze or play dead. “Because of the great diversity of species and their habitats and their predators, there are many different ways of escaping them,” Branco said in November in San Diego at the 2022 meeting of the Society for Neuroscience.
Of course, sometimes, an animal might choose fight over flight. But unless you’re the king of the jungle (or perhaps a roadrunner much smarter than any wily predatory coyote), fighting might be foolish. When an animal is prey, escape is typically its best choice. And it needs to choose fast.
“If it decides to escape, it should make this escape as quickly and accurately as possible,” Branco points out. “And then it should also terminate it as soon as possible because escape is a very costly affair. It costs energy, and it also costs missed opportunities.”
The escape strategy begins with detecting the possible presence of a predator. Detection should be rapid and instinctive — an instant response to sight, sound, or smell. Then, after sensing the threat, an animal’s brain has to quickly implement complex algorithms giving muscles instructions about how to move and where to move to. It’s a complicated decision-making process involving multiple considerations, including the threat’s proximity, environmental circumstances, and the prey’s own condition.
First among the things to consider is the immediacy of the threat. Sometimes there’s time to determine the predator’s identity before taking evasive action. But often, the response must be quicker. A “looming” threat — in which a blobby image on the retina grows rapidly larger — allows no time to lose. Escape should be initiated before the prey knows who the predator is.
“It doesn’t matter if it is an owl or a car or an object,” says Branco. “If it’s coming fast in your direction, you really want to get out of there and think of what it might be afterward.”
Even the simplest animals have evolved rapid escape actions when detecting an immediate threat. Fruit flies, for instance, adjust the position of their legs in order to jump away from a threatening stimulus. Cockroaches scurry rapidly away in a direction roughly opposite that of an approaching predator — but not always precisely the same direction, choosing from three or four possible paths. If the roaches always chose the exact same angle of escape, predators might devise a counterstrategy, Branco points out.
In more complex animals, elaborate brain circuitry has evolved to detect threats and communicate a threat’s presence to the motor systems that direct the muscles to get moving. For a looming stimulus, a nucleus of nerve cells in the midbrain called the optic tectum has served as the prime threat detector since the early days of vertebrate evolution. (In mammals, the analogous brain structure is known as the superior colliculus.) Cells in the retina detecting a rapidly expanding object send signals to the optic tectum or superior colliculus, alerting the brain to an imminent collision. In turn, the tectum/colliculus signals nerve cells to activate muscles. In mammals, those nerve cells reside in the periaqueductal gray or PAG, a structure in the brain stem.
In mice, neural connections between the superior colliculus and PAG are essential for linking threat detection to escape behavior; research has shown. Presenting a large, dark, circular shadow in an otherwise empty arena induces a mouse to immediately flee toward refuge in a small shelter on the arena’s edge. But if the synapses connecting the superior colliculus to the PAG are cut, mice freeze rather than flee when encountering a looming threat, Branco said at the neuroscience meeting.
Subtler threats
For threats not as rapid or obvious as a looming predator, the brain must be attuned to the slightest sensory signals of a possible predator nearby — motion in a shrub or the cracking of a twig, for instance. Such a signal must then be amplified to become the focus of the brain’s attention. And, unlike with looming threats, successful escape might require some intel on the attacker. In these instances, even more, complicated circuitry must facilitate the brain’s reaction. “Immediate escape actions can be relatively simple, but extended escape often relies on processes such as predicting the motion of a predator or performing memory-based navigation,” Branco and coauthor Peter Redgrave write in the 2020 Annual Review of Neuroscience.
Mice exploring their experimental arena apparently rely on memory to direct their movement back toward their shelter when threatened. When an experimenter surreptitiously removes the shelter while the mouse isn’t looking, a threat induces the mouse to quickly run to the spot where the shelter used to be. Apparently, the mouse doesn’t find shelter by looking for it but by remembering where it is supposed to be. So some part of the brain must store that information and then communicate with the superior colliculus to orchestrate commands about which way to run.
Very recent studies suggest that the brain region providing the superior colliculus with that information is the retrosplenial cortex or RSP. It’s a region in the middle of the brain with connections to multiple other brain structures, including the hippocampus (a structure important for memory).
“RSP neurons encode behaviorally important locations, such as landmarks, reward locations, and a variety of spatial features of the environment,” Dario Campaigner, Branco and collaborators write in a paper that first appeared online and now has been published in Nature.
When synapses connecting the RSP to the superior colliculus are blocked, Campaigner and colleagues found the mouse attempts to escape a threat in the wrong direction. In the real world, Branco says, “this would probably be the last error that the mouse makes.”
Of course, many other parts of the brain contribute to an animal’s threat response. Sometimes neural signals might even inhibit escape behavior — a hungry mouse, for instance, might get a message from the hypothalamus suggesting a delay in reacting to a threat in order to get a little more food first. Much remains to be learned about other aspects of brain circuitry that influence escape behavior.
“We have some decent understanding of the neurobiology behind the implementation of some … escape actions,” says Branco. “But there’s a lot of unknowns.”
Fiddler crabs are unusual among arthropods in that their decision to flee from danger hinges on the speed of a potential predator. This may help them detect an attack early enough to scuttle out of danger.
Among the unknowns are some nuances of how certain prey species have evolved to react more effectively to threat signals. Most arthropods, for instance, respond to a looming threat based on how big the blob in their visual field is. Fiddler crabs, though, respond based on how rapidly the size of the looming image changes, researchers from Australia reported recently in Current Biology. Study author Callum Donohue and colleagues noted that attending to speed rather than size allows the crabs to respond when the predator image is still very tiny, enabling a quick escape to their burrow. This finding suggests that lifestyle and environmental factors may influence how different species respond to threat cues, the researchers write.
Branco says that since controlling escape is such an essential brain function, studying it across many species makes it a “powerful model for the study of neuroscience and behavior.” Deeper knowledge of the neurobiology of escape could reveal mental mechanisms that are “generalizable across behaviors across many species,” he says.
After all, escape is just one of many goal-oriented behaviors that animals must master to win the survival-of-the-fittest sweepstakes. Figuring out how brains control escape might very well produce insights into the neurobiology of other survival strategies.
As Branco and Redgrave note in their Annual Review paper, escape is a well-defined behavior, making it plausible to obtain a complete understanding of the biological algorithms controlling it in a variety of species. A detailed understanding of its complexities, they say, “would then provide an entry point for general understanding mechanisms of… how brains generate natural adaptive behaviors.”
This article originally appeared in Knowable Magazine, an independent journalistic endeavor from Annual Reviews. Sign up for the newsletter.