Scientists calculate the total sum of all matter in the universe
By studying the greatest structures in the universe, scientists reveal exactly how much of the universe we still can't fully account for.
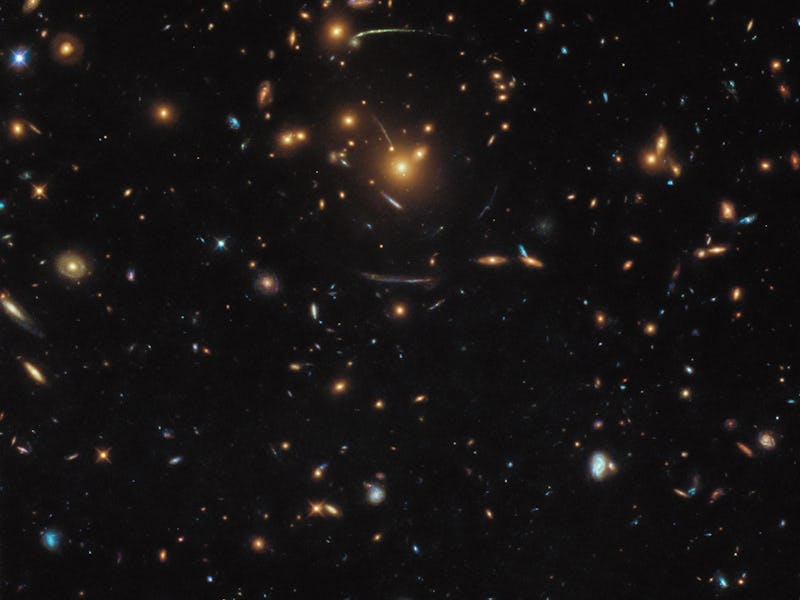
Planets, stars, and other cosmic objects are made up of matter, as are the atoms that exist even in our own bodies. But matter is not all that makes up the universe, and on the cosmic scale it is hard to determine exactly how much is normal matter, and how much is something else.
In a team of scientists from the University of California, Riverside claim they have made the most accurate measurement of the amount of normal matter in the universe — and it is just 31.5 percent.
Their research is detailed in a study published this week in The Astrophysical Journal. The findings could help scientists understand how the universe evolved — and what the rest of it is composed of.
Scientists believe the universe is made up of three things: normal matter, dark matter, and dark energy. Normal matter is the atoms that make up all cosmic objects in the universe, yet it accounts for the smallest proportion of the cosmos.
Astronomers believe that about 20% of the total matter in the universe is made of normal matter, which includes stars, galaxies, atoms, and life, while about 80% is made of dark matter.
In fact, most of the universe is made up of dark energy. Dark energy is theorized to make up some 70 percent of the universe, but despite its abundance, dark energy has never been directly observed nor measured.
Dark matter accounts for the rest of the universe. It is the missing mass that holds all matter, galaxies and stars in place through its gravitational force.
Due to the mysterious and, well, dark nature of dark energy and dark matter, it is hard to determine exactly how much of the universe that they account for.
To calculate the amount of normal matter in the universe, the team behind the new study looked at the largest structures of the cosmos — galaxy clusters.
Galaxy clusters consist of hundreds to thousands of galaxies, bound together through gravity. They form from matter that has collapsed over billions of years under the weight of its own gravity, so the number of clusters observed today correlates to the total amount of matter in the universe.
“A higher percentage of matter would result in more clusters,” Mohamed Abdullah, a graduate student at UCR's department of physics and astronomy, and lead author of the new study, said in a statement. “The ‘Goldilocks’ challenge for our team was to measure the number of clusters and then determine which answer was ‘just right.’"
The team behind the new study created a catalog of galaxy clusters, and compared the number of clusters in their catalog to simulations of clusters to determine the total amount of normal matter. By doing so, they calculated the best combined value of normal matter to be at 31.5 percent of the total amount of matter and energy in the universe.
The remaining 68.5 percent is dark energy, according to the study.
Understanding dark energy is crucial to our understanding of the universe. This dark force is responsible for the accelerating rate of the expansion of the universe, pulling galaxies apart with its strong gravitational force.
As scientists get a better idea of the expansion rate of the universe, they will also get better insight at how the universe evolved over time, and where it all began.
Abstract: We derive cosmological constraints on the matter density, , and the amplitude of fluctuations, , using , a catalog of 1800 galaxy clusters we identified in the Sloan Digital Sky Survey-DR13 spectroscopic data set using our GalWeight technique to determine cluster membership. By analyzing a subsample of 756 clusters in a redshift range of 0.045 ≤ z ≤ 0.125 and virial masses of M ≥ 0.8 × 1014 with mean redshift of z = 0.085, we obtain (systematic) and (systematic), with a cluster normalization relation of . There are several unique aspects to our approach: we use the largest spectroscopic data set currently available, and we assign membership using the GalWeight technique, which we have shown to be very effective at simultaneously maximizing the number of bona fide cluster members while minimizing the number of contaminating interlopers. Moreover, rather than employing scaling relations, we calculate cluster masses individually using the virial mass estimator. Since is a low-redshift cluster catalog we do not need to make any assumptions about evolution either in cosmological parameters or in the properties of the clusters themselves. Our constraints on and are consistent and very competitive with those obtained from non-cluster abundance cosmological probes such as cosmic microwave background, baryonic acoustic oscillation (BAO), and supernovae. The joint analysis of our cluster data with Planck18+BAO+Pantheon gives and .
This article was originally published on