Black holes eat neutron stars for breakfast — and burp out gravitational waves
“In physics, we often say that exceptional discoveries require exceptionally strong evidence.”
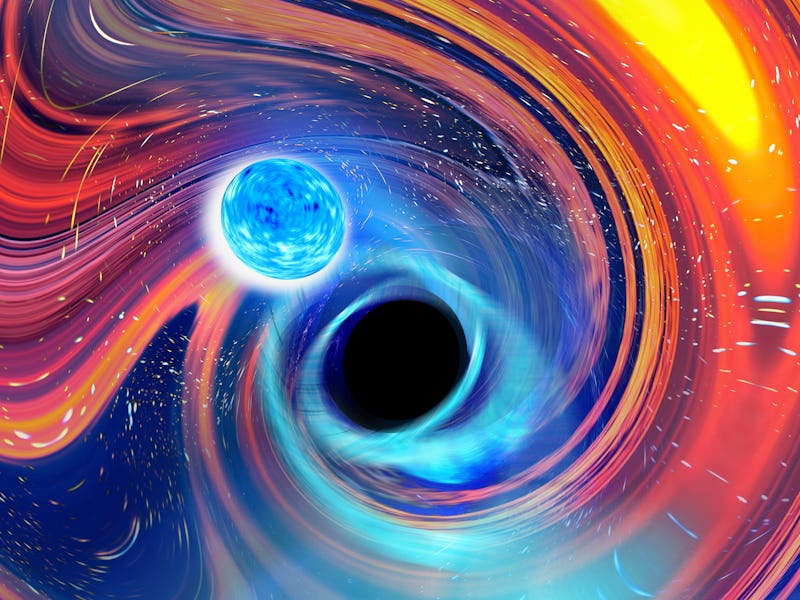
Almost one billion years ago, a black hole sent a neutron star into a death spiral.
And then, some 200 million years later, another black hole claimed yet another victim neutron star with a death spiral.
While “death spiral” might sound like a WWE move, it is actually a scientific term given to a very real, and very metal, phenomenon: Specifically, a death spiral is when a black hole devours a neutron star out in the far reaches of the Universe like a massive Pac-Man munching up a dot.
Both of these death spirals predate the dinosaurs by hundreds of millions of years, yet news of these cosmic collisions only reached Earth in January 2020, in the form of rare, rippling gravitational waves — both within ten days of one another.
“In physics, we often say that exceptional discoveries require exceptionally strong evidence,” physicist Patrick Brady tells Inverse. And in this case, he says, they got it.
What’s new — The first gravitational wave hit our detectors at the Laser Interferometer Gravitational-Wave Observatory (LIGO) in Louisiana and the Virgo gravitational-wave observatory in Italy on January 5, 2020, and another pinged ten days later, on January 15. Together, they represent the dawn of a new era of understanding the until-now elusive black hole-neutron star pair.
The findings, published in the Astrophysical Journal Letters, illuminate some of the mechanisms behind phenomena known as binary systems, which involve two entities orbiting each other.
The detection of a neutron star-black hole binary with gravitational waves described in the paper is the third of its kind known to science. Previously, only binary systems involving two black holes or two neutron stars had been detected.
“These detections can help us understand how these binary systems get together in the first place,” Susan Scott, a co-author on the paper and professor at Australian National University, tells Inverse.
According to Scott, the data lead to more questions than answers.
“Are they thrust together in dense systems for example in the center of a galaxy?” she asks.
“Or do they form in isolation and start out as giant stars and go through their whole life cycles together forming supernova and eventually collapsing into a black hole or a neutron star?”
Artist’s impression of a neutron star and black hole about to merge. Credit: Carl Knox, OzGrav-Swinburne University.
Here’s the background — Astrophysicists had predicted the existence of black hole-neutron star binaries, but the data from the two gravitational waves give them confidence that they found not just one, but two of these elusive pairs.
A neutron star is the collapsed core of a massive, supergiant star. Clocking in at 1.4 solar masses and with a radius of about 10 kilometers, neutron stars are incredibly small and incredibly dense — in fact, they are the most dense stellar object we know of.
Black holes, on the other hand, can vary wildly in size and mass and possess such intense gravity that anything sucked in, including light, cannot escape.
When a black hole meets a neutron star, they essentially form a galactic ballroom dance duo, except at the end of this passionate tango, the neutron star is devoured by the insatiable black hole.
The discovery — The detectors that monitor for gravitational waves use a method called matched filtering, which picks out gravitational wave signals from noise — like how a person in a noisy, crowded room can pick out and listen to one voice at a time.
The scientists knew that the gravitational waves must have come from dense, compact objects far in the Universe — so dense that the two objects must have merged into one, instead of deteriorating. They also knew that the only objects dense enough to be the heavier object in the binary are black holes, and the masses of the second, lighter object appear consistent with that of neutron stars.
The first image of a black hole and its shadow, taken in 2019.
The sheer scarcity of the gravitational waves radiating from these events was, in this case, also a boon for the scientists. One of the binaries detected produces a gravitational wave signal less than once every 100,000 years, so it was fairly unmistakable when it hit the detectors.
“The identification of two different events in which a neutron star collided with a black hole builds our confidence that these signals represent a previously undetected class of mergers,” Brady explains. Brady is the spokesperson for LIGO, as well as a professor at the University of Wisconsin, Milwaukee. He isn’t the only confident one: More than 1,000 scientists contributed to this research.
Why It Matters — Aside from proving that this third kind of binary system can exist, the research also offers clues to the evolution of stellar bodies in the cosmos — from their birth to their death.
Black holes are a mystery to scientists because we still don’t know exactly what happens to something once it gets gulped down.
And neutron stars, which are a star’s end-phase of life, can tell us about star formation and life cycle, and potentially about the origins of the Universe.
“[I]t helps us to understand the life and death of stars throughout the universe,” Scott says.
What’s Next — Scott points to the new questions this research raises — more than enough to keep her and her colleagues at LIGO and VIRGO going through 2022. There are countless things to be detected in our Universe after all, so it’s on to the next phenomenon.
“We are hoping to detect the gravitational waves from a supernova exploding,” says Scott.
But Jess McIver, a LIGO researcher and professor at the University of British Columbia, tells Inverse that this discovery can also help us take note of things happening closer to home, in our own galaxy.
“As we learn more about extragalactic neutron star-black hole binaries from gravitational waves, we'll be able to infer more about the binaries lurking in our own Milky Way galaxy,” she says.
Abstract: We report the observation of gravitational waves from two compact binary coalescences in LIGO’s and Virgo’s third observing run with properties consistent with neutron star–black hole (NSBH) binaries. The two events are named GW200105_162426 and GW200115_042309, abbreviated as GW200105 and GW200115; the first was observed by LIGO Livingston and Virgo and the second by all three LIGO–Virgo detectors. The source of GW200105 has component masses -8.9+1.5 .2 and -1.9+ M 0.20.3, whereas the source of GW200115 has component masses -5.7+2.11.8 and -1.5+ M 0.30.7 (all measurements quoted at the 90% credible level). The probability that the secondary’s mass is below the maximal mass of a neutron star is 89%–96% and 87%–98%, respectively, for GW200105 and GW200115, with the ranges arising from different astrophysical assumptions. The source luminosity distances are -280+110110 and -300+ Mpc 100 150 , respectively. The magnitude of the primary spin of GW200105 is less than 0.23 at the 90% credible level, and its orientation is unconstrained. For GW200115, the primary spin has a negative spin projection onto the orbital angular momentum at 88% probability. We are unable to constrain the spin or tidal deformation of the secondary component for either event. We infer an NSBH merger rate density of -45+ Gpc- yr- 33 75 3 1when assuming that GW200105 and GW200115 are representative of the NSBH population or -130+ Gpc- yr- 69112 3 1under the assumption of a broader distribution of component masses.
This article was originally published on