Scientists predict a pill could turn night owls into early birds
“Time is everything in biology.”
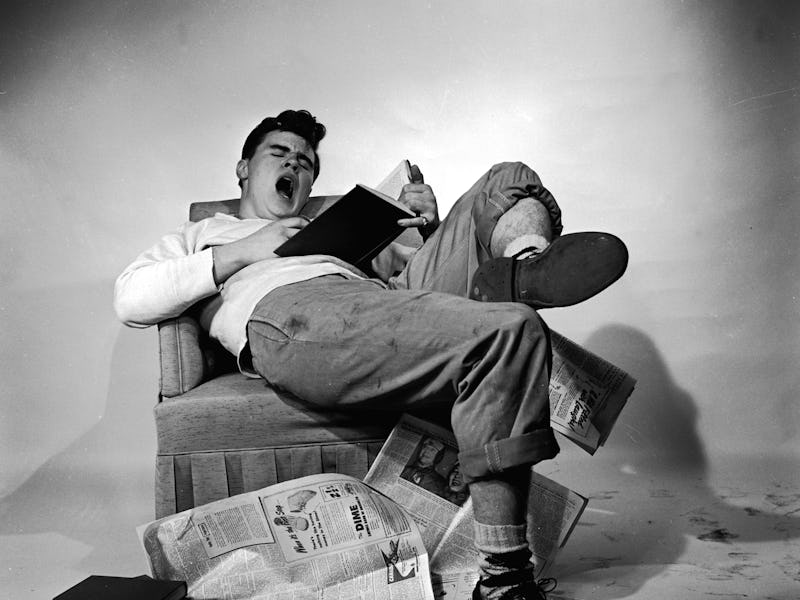
Picture plants that bloom year-round. A plane trip to China followed by no jet lag. Waking up at dawn to easily begin a workday.
In a not-so-distant future, these scenarios could be reality.
In a paper published Tuesday in the journal Applied Physics Reviews, researchers from Pennsylvania and Beijing present a statistical model for identifying the key drivers of our circadian rhythms: clock genes. Critically, this approach allows scientists to pinpoint the complex networks associated with clock genes, which determine how plants, animals, and humans interact biologically with time.
“Based on these networks, we can identify the road map of how the genes interact with other genes to determine the circadian rhythm,” Rongling Wu, the senior author of the study, tells Inverse. Wu is the Director of the Center for Statistical Genetics in the departments of public health sciences and statistics at Pennsylvania State University.
This model (which works in tandem with software) is essentially a tool capable of processing vast quantities of genetic information. And it could potentially pave the way for gene editing or drugs with wide applications — ranging from drugs that could help night owls become early birds to a way to drastically increase the yield of certain crops.
What you need to know first — All living creatures, including people, follow a kind of biological schedule that’s determined by our genes and their response to the environment around us. For most, that cycle lasts around 24 hours (coastal marine mammals operate off a 12-hour cycle).
“... we can identify the road map of how the genes interact with other genes to determine the circadian rhythm.”
People operate off that 24-hour cycle, or circadian rhythm. It’s why we crave sleep when it’s dark and perk up when the sun rises. But it’s more than bedtime — it’s a complex set of events that originates in our DNA and is responsible for the slowing of our heart rate, the production of melatonin, and the blood pressure drop that happens at certain times throughout the night.
Identifying particular genes, gene variations, and how they interact with one another to trigger biological processes, is an elusive goal with enormous potential, argues Wu and colleagues.
For example, identifying the set of gene variations that causes a stalk of wheat to lie dormant instead of growing could allow you to create a super-crop that never rests.
“Time is everything in biology,” Wu says.
Clock genes can be identified with a new tool, and grouped into gene regulatory networks shown here.
How it works — The approach the research team proposes starts with the complete genomes of a large population — all their genetic data — then plugs it into the software.
The complex statistical model, in essence, would crunch the numbers and identify gene networks responsible for certain traits — traits like your blood pressure.
The model is based on the theories of genetic mapping and association studies, which can identify the genetic variants that actually affect a function in a body or a plant. In this case, the genes are those related to circadian rhythms. These are known as “clock quantitative trait loci.”
That’s because “clock genes” are not just one piece of DNA — they may be many different combinations of sequences that affect the different processes in the circadian cycle. And they don’t work in isolation: Certain biological pathways carry environmental signals to them. Once received, more pathways tell the rest of the body to carry out a certain task — like producing a certain protein.
This complex mapping through statistical analysis could reveal the most important genetic markers for circadian processes — or at least, give researchers more precise places in a genetic sequence to study.
“The methods that we developed can capture the whole picture of all genes — all possible genes,” Wu says. “We just proposed a turnkey approach to clock gene identification.”
What's new — Past efforts to identify these critical clock genes have hinged on genetic mutants. These are mutations to an organism’s genome that affects its circadian rhythm.
But these efforts, Wu says, are limited. Though they’ve helped to identify clock genes in a number of species (fruit flies, cyanobacteria, mice) these studies can only single out mutations responsible for a change in circadian regulation by studying them in one genome.
This model could theoretically lead to a pill that could adjust your sleeping habits.
This model, on the other hand, works backward. For example, instead of altering a mouse gene and seeing how it affects an animal in real life, this framework could analyze the traits of a family of mice and map out groups of genes that were potentially responsible for those traits as they relate to a circadian cycle.
The new tool (which is still in its infancy) could also be used to differentiate how clock genes work in different species and even in different tissues of one organism — a novel advance that would allow circadian rhythm scientists to more precisely isolate genes with different functions throughout the body.
Why it matters — This approach to analyzing genes could potentially isolate the genetic networks most responsible for setting our own circadian clocks.
“So if I take that medicine, that medicine activates an early bird gene in the night.”
And once the work of finding the right clock genes is done, you could theoretically develop a drug to express certain traits on command, Wu says.
“[Say] I'm an early bird, but I need to work very late in the night for my job. So if I take that medicine, that medicine activates an early bird gene in the night,” he says. “We could change the ability to make you more active, so you can still keep working in the night.”
It could work the same way for plants that we depend on for food.
“Let’s say we want to produce the correct yield of fruit in tomatoes,” Wu says. “In this case, we may activate the clock gene that is related to flowering and the reproductive process.”
The potential for the tool is expansive, Wu argues. It could be used to help to treat people with disorders related to their circadian rhythm, for example, or even to make a medicine that cures jet lag.
What’s next — Wu says he’s already shared the software that runs the research team’s complex equations with other researchers, who have provided feedback on how well it’s worked in practice. For example, his students ran genetic data from other researchers about a certain type of sea shrimp through the statistical model.
“I had no knowledge about shrimp genetics and their clock genes, but they just simply provided me data,” he said. When he sent the results back to the researchers, who already knew about clock genes in shrimp “they looked at this, and they were very happy — they said it was just what they would have expected.”
Wu was pleased that in a blind test like this, the results matched what was already known. The next step is to make the software more user-friendly, he says — so that a wider community of researchers with genetic data could discover more clock genes.
Abstract: All multicellular organisms embed endogenous circadian oscillators or clocks that rhythmically regulate a wide variety of processes in response to daily environmental cycles. Previous molecular studies using rhythmic mutants for several model systems have identified a set of genes responsible for rhythmic activities and illustrated the molecular mechanisms underlying how disruptions in circadian rhythms are associated with the sort of aberrant cell cycling. However, the wide use of these forward genetic studies is impaired by a limited number of mutations that can be identified or induced only in a single genome, limiting the identification of many other conserved or non-conserved clock genes. Genetic linkage or association mapping provides an unprecedented glimpse into the genome-wide scanning and characterization of genes underlying circadian rhythms. The implementation of sophisticated statistical models into genetic mapping studies can not only identify key clock genes or clock quantitative trait loci (cQTL) but also, more importantly, reveal a complete atlas of the genetic control mechanisms constituted by gene interactomes. Here, we introduce and review an advanced statistical mechanics framework for coalescing all possible clock genes into intricate but well-organized interaction networks that regulate rhythmic cycles. The application of this framework to widely available mapping populations will reshape and further our understanding of the genetic signatures behind circadian rhythms for an enlarged range of species including microbes, plants, and humans.
This article was originally published on