Quantum computing: One of its biggest obstacles has melted
Warming up these quantum bits could bring costs down from millions of dollars to just thousands.
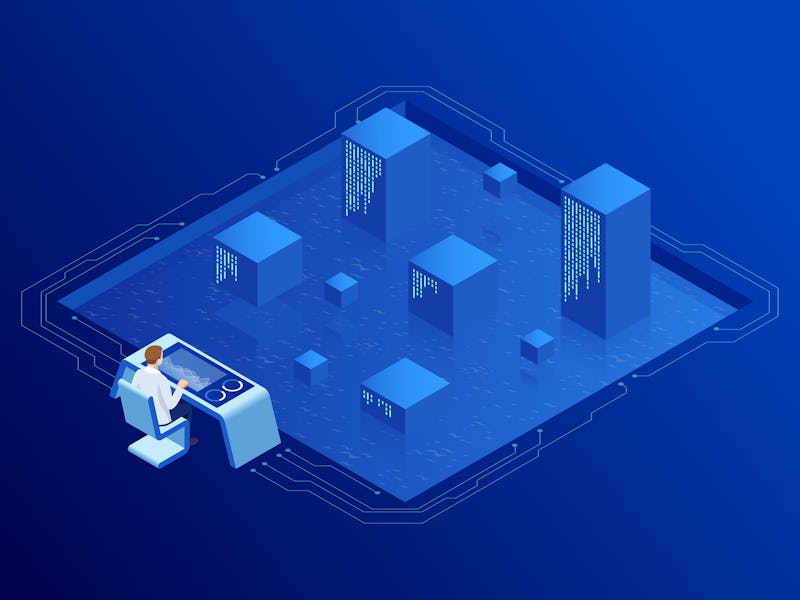
Quantum computing is finally coming in from the cold. Warming up this frozen technology could bring it mainstream like never before.
Despite promises from the likes of Google of quantum computers' supremacy, actually realizing the full computing potential of these computers is easier said than done. In order to even run these machines, they must first be stored in million-dollar fridges designed to keep their supercharged quantum bits, or qubits, at a chilly 1.1 degrees Kelvin (-458 degrees Fahrenheit.) The expense of cooling these superconducting qubits alone makes scaling them to a computationally significant number nearly impossible.
But scientists in two recent Nature studies have found a way to not only warm up these qubits but to scale them using everyday silicon materials instead of superconducting ones.
This innovation would save hundreds of thousands of dollars and could finally bring important quantum computing benefits, like super-secure encryption and precision drug manufacturing, to life.
Published back-to-back this past week in Nature, researchers from the Netherlands and Australia have revealed in two complementary studies a way to design qubits that can operate at temperatures 15 times warmer than existing models.
Andrew Dzurak, a co-author on the first paper and professor nanoelectronics at the University of New South Wales (UNSW) Sydney, said in a statement that while the qubits in their study are still extremely cold, about 1.5 (-457 Fahrenheit) degrees Kelvin, the relatively small temperature increase provides large benefits.
"This is still very cold, but is a temperature that can be achieved using just a few thousand dollars’ worth of refrigeration, rather than the millions of dollars needed to cool chips to 0.1 Kelvin,” says Dzurak. “While difficult to appreciate using our everyday concepts of temperature, this increase is extreme in the quantum world.”
In order to achieve these "hot" qubits, Dzurak and his team designed a processing unit that encased a pair of qubits in silicon. By using electron tunnelling to read the states of these qubit pairs without disturbing them, the team was able to keep their qubit unit stable at the warmer temperatures.
Dzurak said that this result will be incredibly important when it comes to scaling the number of qubits used in quantum computers.
"Every qubit pair added to the system increases the total heat generated,” said Dzurak, “and added heat leads to errors. That’s primarily why current designs need to be kept so close to absolute zero.”
The proof-of-concept design described in their study, as well as a warm quantum circuit design described in the complementary study, would allow for scaling of qubits without sacrificing too much stability. Where today's quantum computers boast only 100s of qubits, Dzurak said a design like this could easily scale to millions.
But that doesn't mean these hot, silicon qubits are without obstacles to overcome. In the study, the researchers write that higher temperatures increase the noise experienced by these qubits, or in other words makes these qubits less stable. Continuing to perfect this design and reduce quantum noise and errors will be essential to ensure the successful scaling of these silicon qubits.
"We've shown that silicon qubits work just fine at higher temperatures, meaning we can now think about building quantum computers will millions of qubits," says Dzurak in a YouTube video describing the study. "We've already designed such a chip based on the same silicon technology used to manufacture conventional computer chips. And when built, this will open the way to solve problems of global significance."
Abstract: Quantum computers are expected to outperform conventional computers in several important applications, from molecular simulation to search algorithms, once they can be scaled up to large numbers—typically millions—of quantum bits (qubits). For most solid-state qubit technologies—for example, those using superconducting circuits or semiconductor spins—scaling poses a considerable challenge because every additional qubit increases the heat generated, whereas the cooling power of dilution refrigerators is severely limited at their operating temperature (less than 100 millikelvin). Here we demonstrate the operation of a scalable silicon quantum processor unit cell comprising two qubits confined to quantum dots at about 1.5 kelvin. We achieve this by isolating the quantum dots from the electron reservoir, and then initializing and reading the qubits solely via tunnelling of electrons between the two quantum dots. We coherently control the qubits using electrically driven spin resonance in isotopically enriched silicon 28Si, attaining single-qubit gate fidelities of 98.6 per cent and a coherence time of 2 microseconds during ‘hot’ operation, comparable to those of spin qubits in natural silicon at millikelvin temperatures. Furthermore, we show that the unit cell can be operated at magnetic fields as low as 0.1 tesla, corresponding to a qubit control frequency of 3.5 gigahertz, where the qubit energy is well below the thermal energy. The unit cell constitutes the core building block of a full-scale silicon quantum computer and satisfies layout constraints required by error-correction architectures. Our work indicates that a spin-based quantum computer could be operated at increased temperatures in a simple pumped 4He system (which provides cooling power orders of magnitude higher than that of dilution refrigerators), thus potentially enabling the integration of classical control electronics with the qubit array.