Bizarre, never-before-seen material could drive the next-gen of computers
Scientist uses soccer to explain how super-rare material will change computing.
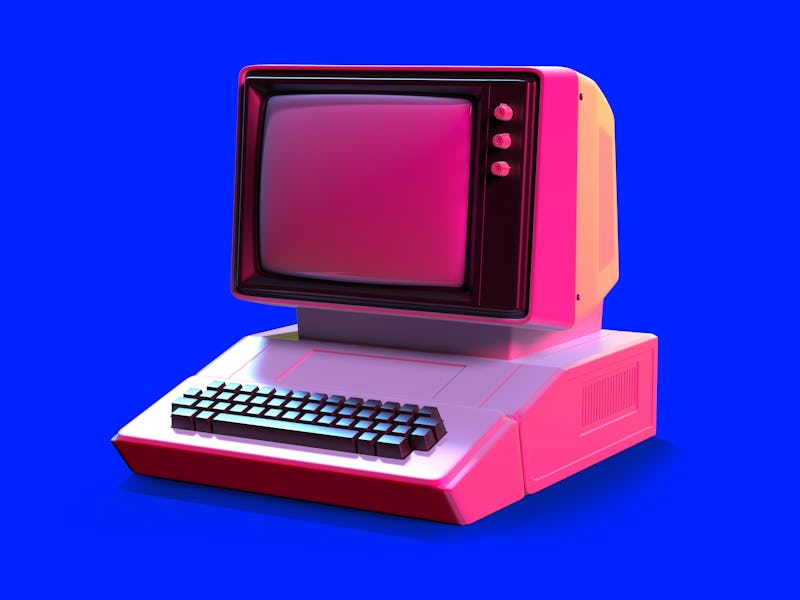
Technology companies constantly roll out their "next-generation" phones and computers with the promise that they're better than ever before. But iPhone updates can't quite compare to what a new study predicts: A next-generation computer made with an ultra-rare, never-before-seen material.
After more than 30 years of searching, an international team of physicists and chemists discovered a material with a trifecta of rare and highly sought after characteristics. This material, KV3Sb5, has the potential to revolutionize the design of computer memory and processors — and is shaking up fundamental understandings of physics.
This finding was published Friday in the journal Science Advances. The discovery involves the documentation of a "giant" electromagnetic effect in an already complicated and rare material.
Mazhar Ali is the study's senior author and a researcher at the Max Planck Institute of Microstructure Physics. Ali tells Inverse that KV3Sb5 is something called a "metallic frustrated magnet."
A material like this has been highly sought after for around 30 years because "theorists have speculated that the interplay of frustrated magnetism with traveling electrons could result in exotic properties like unconventional superconductivity and more," Ali says.
"Metallic frustrated magnets are very, very rare," he explains.
KV3Sb5's oddness didn't stop there: It also houses a special kind of electron called a "Dirac electron," Ali says, which means its electrons are both much faster and way lighter than your run-of-the-mill electron.
Coupled with the material's malleability — it's easy to flake into individual layers and cooperative during fabrication — and it becomes one of a kind.
"There is no other example material with this combination of traits," Ali remarks.
How does it work — The giant electromagnetic effect that these combined characteristics make possible is something called the anomalous Hall effect (AHE.) This effect refers to a way magnets and charge interact and can either be intrinsic (meaning the structure of the material determines how electrons move through it) or extrinsic (in which certain features of the structure create more scattering).
Either of these AHEs will change how electrons scatter off the material, thus changing out information is carried.
"There is no other example material with this combination of traits."
To explain what exactly that looks like inside this material, Ali says we can turn to soccer for an analogy.
"Intrinsic is like if Christiano Ronaldo was making a curved pass around some defenders, without colliding with them, by kicking the soccer ball [the electron] in a special way," Ali says.
"Extrinsic is like the ball bouncing off of a defender — aka an electron off of a magnetic scattering center —and going to the side after the collision. Most extrinsically dominated materials have a random arrangement of defenders on the field — [like the] scattering centers randomly situated throughout the crystal."
Ali explains that most extrinsically arranged materials have these defenders scattered randomly through the field (or material). KV3Sb5, on the other hand, plays a tighter defense.
"KV3Sb5 belongs to a class of materials known as cluster magnets," Ali says. "It has defenders grouped together and arranged on the field in a special pattern... In this scenario, the ball scatters off of the cluster of defenders, rather than a single one, and is more likely to go to the side than if just one was in the way."
In KV3Sb5, this pattern is a triangle of 3 magnetic scattering centers. This is thought to underly a recently proposed spin-cluster skew scattering mechanisms linked to AHE, which, Ali says "demonstrated for the first time in this material; because it has the right ingredients to host this effect."
And, because the electrons at play in this material are super-fast Dirac electrons, Ali says this is equivalent to Ronaldo kicking the ball instead of a 10-year-old. The result? A giant AHE.
How can it be used — But what can you do with a material so special? One option exciting scientists is as a replacement for platinum in computing and memory technology.
"The same physics that governs this AHE should also drive the spin Hall effect, where instead an electron gaining the orthogonal velocity, it is just the electron's spin," Ali explains. "Large spin Hall effects in metals are highly sought after for spintronic applications like next generation computation and memory technology."
This type of technology, Ali says, is already commercially available — this IBM and Everspin — but these technologies are based on Platinum. He explains that finding a cheap and stable alternative to platinum would "be a big win" — and this finding could make that possible.
Another exciting avenue for physicists to explore, says Ali, is whether or not this material could superconduct at low temperatures -- a trait that, when combined with other components, would benefit the future of quantum computing as well.
Where there's one super weird, rare material, they hope to find another. By further exploring this material and those like it, scientists aim to learn more about this fundamental physics phenomena.
Abstract: The anomalous Hall effect (AHE) is one of the most fundamental phenomena in physics. In the highly conductive regime, ferromagnetic metals have been the focus of past research. Here, we report a giant extrinsic AHE in KV3Sb5, an exfoliable, highly conductive semimetal with Dirac quasiparticles and a vanadium Kagome net. Even without report of long range magnetic order, the anomalous Hall conductivity reaches 15,507 Ω−1 cm−1 with an anomalous Hall ratio of ≈ 1.8%; an order of magnitude larger than Fe. Defying theoretical expectations, KV3Sb5 shows enhanced skew scattering that scales quadratically, not linearly, with the longitudinal conductivity, possibly arising from the combination of highly conductive Dirac quasiparticles with a frustrated magnetic sublattice. This allows the possibility of reaching an anomalous Hall angle of 90° in metals. This observation raises fundamental questions about AHEs and opens new frontiers for AHE and spin Hall effect exploration, particularly in metallic frustrated magnets.