Microscopic robots could take your body on a fantastic voyage
Scientists have been developing microrobots that could imitate white blood cells. Injecting them into the bloodstream to help fight cancer could be next.
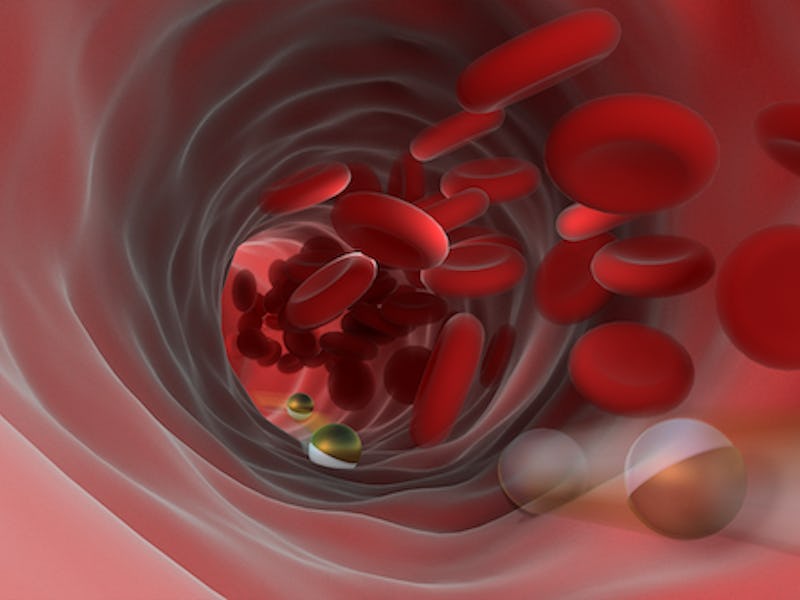
Tiny robots that operate within the human body have long been in the realm of science fiction, from Fantastic Voyage to The Magic School Bus. Scientists at the Max Planck Institute for Intelligent Systems in Stuttgart, Germany have crossed into the world of science fact with microrobots built to resemble white blood cells. Working with the body, these robots could revolutionize minimally invasive medical treatments.
Their findings were published in Science Robotics on May 20, titled “Multifunctional surface microrollers for targeted drug delivery in physiological blood flow”.
Testing out their robots in an artificial bloodstream, scientists were able to make sure their robots could replicate the movements of white blood cells.
To understand these robots, it’s worth understanding one of the body’s crucial lines of defense in defending itself. Formally known as leukocytes, these cells are made in bone marrow and have any number of means to attack invading germs. They can break down bacteria, digest bacteria, create histamines to identify bacteria, or create more antibodies to fight off disease.
How the movies imagined life inside the blood stream.
The bloodstream is no easier place—to withstand the pressures of bloodflow, the robots had to move at speeds of 600 micrometers per second, which adjusted for size comes out to 76 body lengths per second. According to the Institute, this speed makes theirs the fastest magnetic microrobot at this size scale.
Each robot, made out of glass microparticles, has a diameter of under 8 micrometers. With gold film and thin nickel on one side, the other is filled with anti-cancer drug molecules known as doxorubicin and specific biomolecules that can recognize cancer cells known as anti-HER2.
Yunus Alapan, a postdoctoral researcher in the Physical Intelligence Department and the co-lead author, explains in a press statement.
“Using magnetic fields, our microrobots can navigate upstream through a simulated blood vessel, which is challenging due to the strong blood flow and dense cellular environment. None of the current microrobots can withstand this stream. Additionally, our robots can autonomously recognize cells of interest such as cancer cells. They do this thanks to a coating of cell-specific antibodies on their surface. They can then release the drug molecules while on the move.
An artist's representation of the robots (lower right corner) entering the bloodstream.
While each robot can only carry small amounts of doxorubicin and anti-HER2, as a swarm they could be the cavalry coming in to relieve weary leukocytes. That’s the next goal, says co-author and Ph.D student Ugur Bozuyuk.
Therapeutic cargoes transported by a single microrobot would not be sufficient, given the size difference between a microrobot (around 10 micrometers) and the target tissues (thousands of micrometers). Therefore, the controlled manipulation of a high number of microrobots in a swarm would be necessary to generate a sufficient effect. But we are still far from that,” Bozuyuk says.
The inspiration for the robots came not from science fiction, but rather one of the most impactful speeches in the history of 20th century technology. In 1959, physicist Richard Feynmann gave a lecture at the annual conference of the American Physical Society titled “There’s Plenty of Room at The Bottom.” During this speech, Feynmann threw down the gauntlet in the form of two $10,000 prizes in order to generate excitement for making technologies smaller. The speech is widely credited with helping to kickstart the widespread development of nanotechnology.
Richard Feynman, the famed physicist whose speech kickstarted the nanotech revolution that began in the 1980s and continues today.
“A biological system can be exceedingly small,” Feynmann said at the time. “Many of the cells are very tiny, but they are very active; they manufacture various substances; they walk around; they wiggle; and they do all kinds of marvelous things – all on a very small scale. Also, they store information. Consider the possibility that we too can make a thing very small which does what we want – that we can manufacture an object that maneuvers at that level!”
Both of Feynmann’s direct challenges—to create the world’s tiniest motor and to write the equivalent of the Encyclopedia Britannica on the head of a pin—were eventually met. As the Institute’s microrobots progress into swarms, it’s possible that one of his metaphors could become reality.
Abstract: Mobile microrobots offer great promise for minimally invasive targeted medical theranostic applications at hard-to-access regions inside the human body. The circulatory system represents the ideal route for navigation; however, blood flow impairs propulsion of microrobots especially for the ones with overall sizes less than 10 micrometers. Moreover, cell- and tissue-specific targeting is required for efficient recognition of disease sites and long-term preservation of microrobots under dynamic flow conditions. Here, we report cell-sized multifunctional surface microrollers with ~3.0 and ~7.8-micrometer diameters, inspired by leukocytes in the circulatory system, for targeted drug delivery into specific cells and controlled navigation inside blood flow. The leukocyte-inspired spherical microrollers are composed of magnetically responsive Janus microparticles functionalized with targeting antibodies against cancer cells (anti-HER2) and light-cleavable cancer drug molecules (doxorubicin). Magnetic propulsion and steering of the microrollers resulted in translational motion speeds up to 600 micrometers per second, around 76 body lengths per second. Targeting cancer cells among a heterogeneous cell population was demonstrated by active propulsion and steering of the microrollers over the cell monolayers. The multifunctional microrollers were propelled against physiologically relevant blood flow (up to 2.5 dynes per square centimeter) on planar and endothelialized microchannels. Furthermore, the microrollers generated sufficient upstream propulsion to locomote on inclined three-dimensional surfaces in physiologically relevant blood flow. The multifunctional microroller platform described here presents a bioinspired approach toward in vivo controlled propulsion, navigation, and targeted active cargo delivery in the circulatory system.