Scientists at LIGO are one step closer to solving general relativity’s biggest problem
Scientists are one step closer to solving general relativity’s biggest problem.
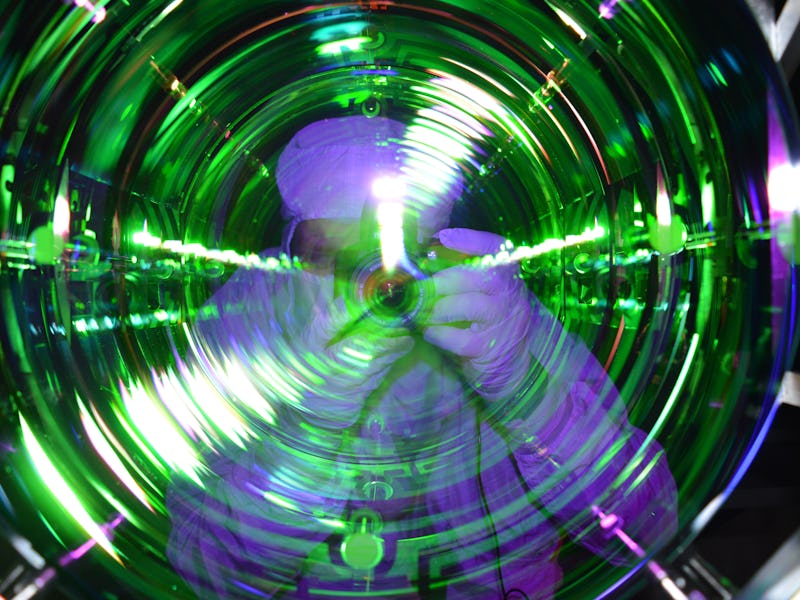
Just shy of a century after Einstein penned his infamous paper on general relativity scientists finally confirmed a cornerstone of his predictions in 2015 — gravitational waves, little curvatures of gravity woven into spacetime were real and we can “feel” them.
To do this, scientists used a new kind of observatory called LIGO (Laser Interferometer Gravitational-wave Observatory) that is fine-tuned to hunt for small disturbances in the fabric of spacetime caused by cosmic collisions, like black hole or neutron star mergers.
But this is only just the beginning of what LIGO can do, a team of international researchers reports in a new study published Thursday in the journal Science. Using new techniques to quantum cool LIGO’s mirrors, the team says that LIGO may soon also help them understand the quantum states of human-sized objects instead of just subatomic particles.
Vivishek Sudhir is a coauthor on the paper and assistant professor of mechanical engineering at the Massachusetts Institute of Technology. He tells Inverse that physicists have long theorized that gravity may be the culprit behind why large items don’t exhibit quantum behavior.
“One way to test this theory is by an experiment where one realizes a quantum state of an object that is also massive enough that the effect of gravity on it can be measured,” Sudhir explains.
In this new experiment, the LIGO instrument is both large enough and cold enough to test this out in practice.
One of LIGO’s four 40-kg mirrors cooled during the experiment. Together, these mirrors form a mechanical oscillator of 10-kg — which the team cooled down in their experiment.
What’s new — When scientists talk about or study quantum phenomena they’re nearly always talking about incredibly tiny particles — much, much smaller than the human eye could see. At this small scale, it’s possible to observe quantum phenomena (like quantum entanglement where physically separated particles will still respond in tandem to stimuli.)
But these same kinds of phenomena aren’t so eager to be observed in more human-sized objects, explain the authors. Due in part to objects’ thermal environment masking these delicate quantum signals.
“The motion of a mechanical object, even a human-sized object, should be governed by the rules of quantum mechanics,” the authors write in their report. “Coaxing them into a quantum state is, however, difficult.”
This thermal disruption, however, can be overcome by bringing temperatures way down. This is why quantum computers are supercooled, for example. In their new paper the team reports cooling down the largest mass yet — a 10-kilogram mechanical oscillator within LIGO responsible for supporting the mirrors— from room temperature to 77 nanokelvins. This is roughly -459 degrees Fahrenheit and very close to reaching absolute zero.
Temperatures this cold can also be used to create strange, news states of matter — like Bose-Einstein Condensates, which can be used in the detection of gravitational fields.
Why it matters — This mass is 13 times larger than any previous objects that have been cooled to this level the authors report, and could be a huge step toward not only improving the sensitivity of LIGO itself but to exploring other macroscopic quantum phenomena as well.
Such human-sized quantum mysteries could help bring physicists closer to their Holy Grail — a theory to finally explain the discrepancies between the invisible quantum reality and our own.
To avoid contaminating the mirrors, the research team wore cleanroom suits during the experimental procedures.
What is LIGO?
Unlike other astronomical observatories, which collect starlight through round lenses, LIGO is what CalTech calls a “blind” observatory, meaning it doesn’t need light to make its observations. LIGO is broken up into two separate observatory sites — one in Washington state and one in Louisana — that both feature a super-sensitive instrument called a Michelson interferometer.
Here’s what happens when a gravitational wave hits LIGO, according to NASA:
- A gravitational wave caused by a cosmic collision passes by Earth
- As it passes by, it squeezes and stretches space
- LIGO has two outstretched arms (each 4 kilometers long) that cross each other asymmetrically — this is the Michelson Interferometer
- This stretching of space causes LIGOs arms to change in length slightly, which it detects using lasers and reflecting mirrors
Using lasers, LIGO is fine-tuned to detect small disturbances in space-time — like gravitational waves.
Michelson interferometers can be small enough to fit inside a lab but in LIGOs case it is larger than a football field and covered in steel and concrete.
In addition to detecting gravitational waves in 2015, LIGO has also helped scientists detect a “kilonova” (i.e. a black hole merger) in 2017.
What they did — When it comes to actually cooling an object down to near absolute zero — which also corresponds to its lowest-energy or ground state — Sudhir says that the team faced a “chicken and the egg” problem.
“If you need to cool [something] down, you need to extract energy from the object and do that faster than the rate at which energy gets into the object from its surroundings,” says Sudhir.
“However as the object gets colder, smaller perturbations of energy from the surrounding can cause a proportionately larger injection of energy into the object. So, as you get colder, you need to be extracting energy even faster to even be able to maintain that temperature.”
This is complicated further by first needing a cooler object to extract energy in the first place. To solve this problem, Sudhir says their team used something called “feedback cooling.”
“The idea is to use lasers to monitor very precisely how the object jiggles around in response to energy coming in from the surroundings, and then use that information to immediately cancel out that random motion — somewhat like a noise-canceling headphone,” says Sudhir.
In the future, experiments like this could help scientists finally understand how gravity affects quantum mechanics.
Using this technique, the researchers were able to cool the 10-kilogram oscillator down to a temperature that Sudhir says could be used to help detect the effects of gravity on quantum mechanics.
What’s next — While the team’s results are incredibly promising, Sudhir says that future incarnations of LIGO — which would have a 40 km long interferometer and cryogenically cooled mirrors — will be an even greater asset to this exploration.
Ultimately, Sudhir hopes that experiments like these can bring him one step closer to answering questions that he once puzzled over as a young physics student.
“Some of the questions that are probed by these experiments are fairly fundamental — in fact questions that most students of physics might be prompted to ask when they encounter quantum physics for the first time,” he says. “I did too, a decade ago; now I get the chance to ask nature the same question through an experiment.”
Abstract: The motion of a mechanical object, even a human-sized object, should be governed by the rules of quantum mechanics. Coaxing them into a quantum state is, however, difficult because the thermal environment masks any quantum signature of the object’s motion. The thermal environment also masks the effects of proposed modifications of quantum mechanics at large mass scales. We prepared the center-of-mass motion of a 10-kilogram mechanical oscillator in a state with an average phonon occupation of 10.8. The reduction in temperature, from room temperature to 77 nanokelvin, is commensurate with an 11 orders-of-magnitude suppression of quantum back-action by feedback and a 13 orders-of-magnitude increase in the mass of an object prepared close to its motional ground state. Our approach will enable the possibility of probing gravity on massive quantum systems.
This article was originally published on