Scientists want to turn carbon dioxide into plastic. They're getting closer.
A new catalyst could "transform" the economics of CO2 conversion.
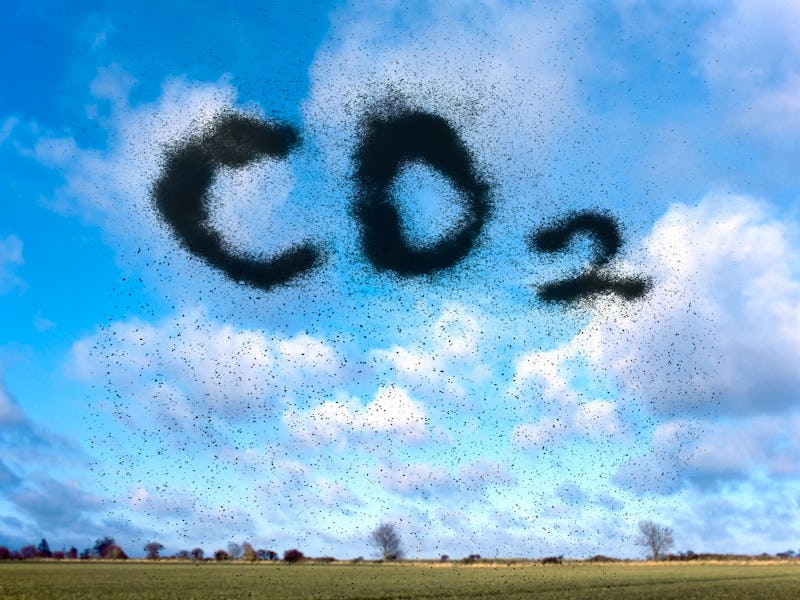
It’s no mystery that CO2 emitted from our cars, homes, and factories are causing near irreversible damage to our environment, and that inspiring greener practices can be hard without economic incentives. But that might be getting easier, say researchers, with the introduction of a new, economically efficient catalyst designed to convert CO2 into the manufacturing chemical ethylene, which is used in the creation of consumer plastic products ranging from medical devices to synthetic fabrics.
The research, published Wednesday in the journal Nature, is the follow-up to a study published last year that described how a copper-based catalyst could convert CO2 into the industrial chemical ethylene. While the first paper demonstrated how this conversion reaction could take place, there were still significant drawbacks that needed to be addressed. Namely, that the catalyst had to be submerged in a chemically basic media to operate (which was wasting time and resources to maintain) and that in addition to ethylene the reaction was also creating unnecessary side products, like carbonate, that would simply dissolve after the reaction had finished.
Together these challenges meant that the catalyst wasn’t cost-efficient, lead author of the new paper and post-doctoral fellow, Fengwang Li, said in a statement.
“One of the challenges with this reaction is that while some of the CO2 is converted into ethylene, most of it turns into side products, especially carbonate, which dissolves on the liquid side of the electrolyzer,” said Li. “This undesired loss increases the cost of ensuing product separation and purification.”
Coauthor Fengwang Li (above) demonstrates the copper-based catalyst that the team designed for CO2 conversion.
In this follow-up study, the authors set out to determine how they could improve the efficiency of the catalyst and bring down the cost. To do this they looked at ways to fine-tune the catalyst by introducing a thin film made of a class of molecules called “arylpyridiniums.” The team sifted through dozens of these different arylpyridiniums in trials in order to determine which might have the best — if any — outcome on the catalyst’s performance.
The team was excited to find that the addition of this thin layer of one of these molecules types to the top of the catalyst drastically improved the stability of the catalyst — increasing its stability to nearly 200 hours of operation — and its sensitivity to creating ethylene instead of unnecessary byproducts. The researchers also found that this new film made it possible for the catalyst to perform in a neutral pH media, meaning they no longer had to use costly chemicals to maintain a basic environment.
“The previous system required the water side of the reaction to be at high pH, very basic conditions,” Li said. “But the reaction of the CO2 with caustic soda in the water lowers the pH, so we would’ve had to continuously add chemicals to keep the pH up. The new system works just as well under neutral conditions, so we can eliminate that additional cost, as well as loss of CO2 in the form of carbonate.”
Lowered cost of maintaining the solution as well as the increased efficiency of the catalyst both spell higher economic value, says University of Toronto Engineering professor and lead on the project, Ted Sargent, and that means more incentive to actually begin converting CO2.
“CO2 has low economic value, which reduces the incentive to capture it before it enters the atmosphere,” Sargent said in a statement. “Converting it into ethylene, one of the most widely-used industrial chemicals in the world, transforms the economics. Renewable ethylene provides a route to displacing the fossil fuels that are currently the primary feedstock for this chemical.”
By increasing the economic value of CO2 through an easier path the ethylene conversion, Sargent hopes that such techniques will begin to become more appealing to industry than continuing to extract more oil to make these industrial plastics.
The catalyst is still a long way away from commercialization. In particular, a challenge going forward will be to continue to scale the prototype without losing efficiency. The team, who already scaled the catalyst prototype in this paper to 5 times the size of the prototype from their previous paper, has already demonstrated how this might be possible, but the task will now be to keep up the pace.
Abstract:
The electrocatalytic carbon dioxide (CO2) reduction reaction (CO2RR) to value-added fuels and feedstocks provides a sustainable and carbon-neutral approach to the storage of intermittent renewable electricity1. The highly selective generation of economically desirable C2 products such as ethylene from CO2RR remains a challenge2. Tuning the stabilities of intermediates to favour a desired reaction pathway offers the opportunity to enhance selectivity3–5, and this has recently been explored on copper (Cu) via control over morphology6, grain boundaries7, facets8, oxidation state9 and dopants10. Unfortunately, the Faradaic efficiency for ethylene is still low in neutral media (60 per cent at a partial current density of 7 mA cm−2 in the best catalyst reported so far9), resulting in a low energy efficiency. Here we present a molecular tuning strategy—the functionalization of the surface of electrocatalysts with organic molecules—that stabilizes intermediates for enhanced CO2RR to ethylene. Using electrochemical, operando/in situ spectroscopic and computational studies, we investigate the influence of a library of molecules, derived via electro-dimerization of arylpyridiniums11, on Cu. We find that the adhered molecules improve the stabilization of an atop-bound CO intermediate, thereby favouring further reduction to ethylene. As a result of this strategy, we report the CO2RR to ethylene with a Faradaic efficiency of 72 per cent at a partial current density of 230 mA cm−2 in a liquid-electrolyte flow cell in neutral medium. We report stable ethylene electrosynthesis for 190 hours in a membrane-electrode-assembly-based system that provides a full-cell energy efficiency of 20 per cent. These findings indicate how molecular strategies can complement heterogeneous catalysts by stabilizing intermediates via local molecular tuning.